You are here
Astronomy through the back door

(This interview was originally published in French in issue 15 of the CNRS journal Carnets de Science(link is external))
You are a world-renowned scientist, and one of those who first proposed the Nice model. Could you describe this model to us?
Alessandro Morbidelli1: The Nice model was initially developed in 2005. It is a scenario for the dynamics of the most recent stage in the formation of the Solar System, when it acquired the structure it has today. It introduces the notion of phases of dynamical instability. The model's central idea is based on the presence and mobility of giant planets such as Jupiter and Saturn during the formation of planetary systems. Over time, their orbits change and become transformed, because they are too unstable. The giant planets interact with one another and, in so doing, have a powerful effect on the rest of the Solar System. When they pass close to populations of small bodies, they disrupt their orbits and scatter them.

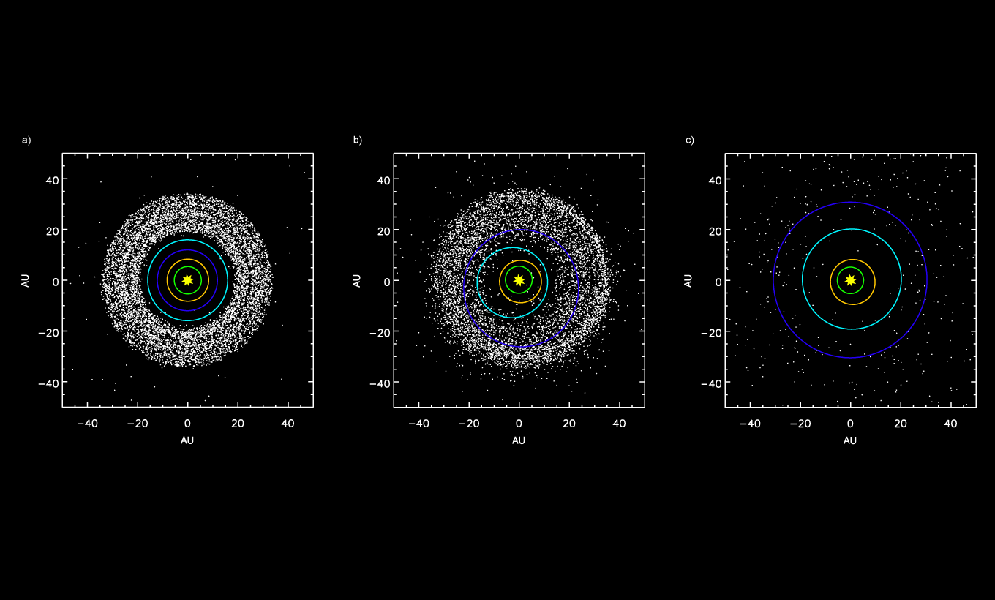
But as soon as you have instability, you also have chaotic phases. When we ran our model, we would sometimes lose an ice giant (Neptune or Uranus), and occasionally even end up with the gas giants (Jupiter and Saturn, Editor's note) in eccentric orbits. Roughly speaking, in 5 to 10% of cases we would land on our feet with a structure similar to our Solar System. However, in absolute terms, a model that tries to replicate this structure with such a low success rate is not robust enough to be convincing. It was the discovery of the first exoplanet in 1995 that confirmed our model. From then on, we knew that planetary systems could display a wide range of structures. Since then, the Nice model has become widely accepted, and has even been refined.
But how do planetary systems form?
A. M.: It all starts with a cloud of matter which, as it collapses under its own weight, forms a star. The remaining matter that isn't dragged down in the collapse forms a disc around the new star, called the protoplanetary disc, which will eventually give rise to the planets that make up the planetary system. The dust contained in this cloud of left-over matter starts to clump together, initially forming small bodies such as comets and asteroids, which in turn begin to collide with one another, generating larger bodies that will become planets.

The standard theory postulates that every planet was born exactly where it is found today. So, the idea that these celestial bodies formed in orbits that were different from the ones they're in today was revolutionary. However, it was already known that the giant planets were the first to come into existence, in a process that took less than 10 million years. As they formed, they accreted part of the helium and hydrogen remaining in the system. As for the rocky planets (Mercury, Venus, Earth and Mars), geochemical data collected on Earth suggest that they emerged 100 million years later.
How did you come to model the formation of planetary systems?
A. M.: After completing a master's degree in physics at the University of Milan, not without some ups and downs, it was in Namur (Belgium) that my research career really began. There, I was welcomed by an extremely amiable professor, Jacques Henrard, who introduced me to chaos theory, in other words, the theory of perturbations. Scientifically speaking, I found myself at the interface between physics and mathematics, and to some extent, a bit removed from my passion for astrophysics. But I was finally able to turn towards astronomy thanks to the numerous applications of chaos theory, which I decided to apply to celestial mechanics. It was then that I started to study the effects of the perturbations of Jupiter on asteroids.
It was this research that drew attention to me, so to speak. My work was noticed by researchers working at the OCA observatory, in Nice (southeastern France), who at that time, in the early 1990s, were running the first numerical simulations in a bid to understand how meteorites could be displaced from the asteroid belt (located between the orbits of Mars and Jupiter, Editor's note) towards Earth.
Back then, the researchers at the Observatory were witnessing some odd phenomena. When they modelled the motion of asteroids, they couldn't figure out why the stable orbits of some objects would suddenly become distorted. They even put it down to computation errors. That was in 1992, and since I had already worked on chaotic dynamics and effects, the researchers at the OCA offered me a postdoctoral fellowship so that I could analyse their simulations, check whether they were correct and, above all, find out what was causing the behaviour they were observing. In a nutshell, I got into astronomy through the back door. The following year I obtained a position in astrophysics at the CNRS. That was the start of a long adventure.
At that time, what state was astrophysics in?
A. M.: It was then that we were receiving the first images of the giant planets and their moons, sent back by the Voyager 1 and 2 spacecraft. We were right at the beginning of the space exploration of the outer Solar System, and this led to two major revolutions. The first was in 1992 with the discovery of the Kuiper belt, a population of small objects lying beyond Neptune. It was almost as if the known Solar System had doubled in size. We could now begin to explore a region containing the remnants of our system’s origins. Of course, at the time we knew nothing about exoplanets.
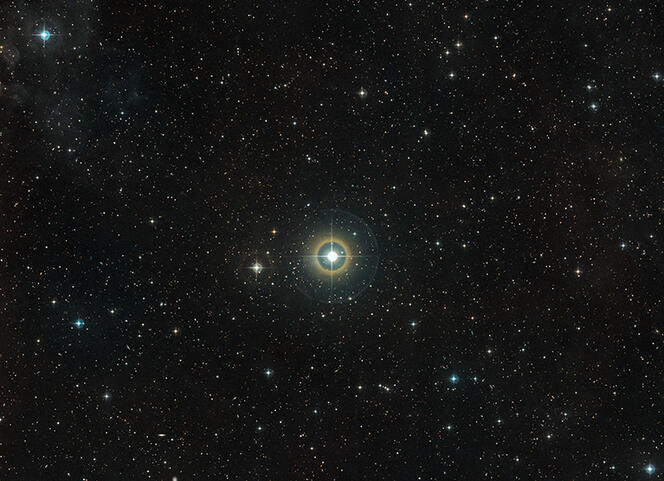
The second revolution came in 1995, with Didier Queloz and Michel Mayor's discovery of the first exoplanet, 51 Pegasi B. Both findings, the Kuiper belt and exoplanets, transformed planetary science and opened up new fields of astronomical research. Today, I'm still astounded by direct images of exoplanets orbiting their stars, or by the close-up pictures of the Kuiper belt object Arrokoth captured by the New Horizons spacecraft. I never thought I'd ever get to see this with my own eyes.
In what ways did the discovery of the first exoplanet transform your research and shake up everything astrophysicists thought they knew?
A. M.: It changed everything! As is the case every time something really new is discovered, it caused a certain amount of panic. Suddenly yoù have a new field of research, you have to dive into it, you have to rethink everything, and your comfort zone is turned upside down. On the other hand, new findings like this are inspiring.
The detection of exoplanets was of fundamental importance because it very quickly raised awareness that other planetary systems were quite different from our own. In this instance, the first extrasolar planet observed was a kind of Jupiter orbiting very close to its star, at a distance similar to that of Mercury to the Sun (around 58 million km, Editor's note). It was totally unexpected! The paradigm then was based solely on the structure of our own Solar System: rocky planets close to their star and gas giants further out.
Following these observations, we rapidly realised that our Solar System was really an exception. At that time, in order to be accepted by the astrophysics community, any model of the evolution of the Solar System had to operate in an almost deterministic way. Models were supposed to be able to reproduce the Solar System every time. But we just couldn’t do it. From the moment the first exoplanets were discovered, it became clear that planetary systems were extremely diverse. Models could no longer be expected to systematically come up with any that were similar in every way to our own. This was a major paradigm shift, and it enabled the Nice model to be favourably regarded by astrophysicists.
What made you decide to become a planetary scientist?
A. M.: I must have been 5 or 6 years old when I discovered the night sky. In those days, our family used to go on holiday to a little village in the mountains overlooking Lake Garda, in the Italian Alps. I used to love going out in the garden at night to play with the shadows. It was a way of overcoming my fear. And the longer I stayed outside, the more my eyes got used to the surrounding darkness. Until one day, I looked up at the sky and saw the Milky Way. Something almost unimaginable today (due to light pollution, Editor's note), but back then you really could see it. And I remember vividly going back into the house to call my father and ask him what it was. He came back outside with me and, even though he was a chemist, he was able to explain the wonderful sight we beheld.

Later, my parents gave me a small second-hand 114 mm telescope for Christmas that I used to take on holiday to observe the stars. I also used it in the city, where observation conditions were far from ideal. Nonetheless, I discovered that in Milan, where I lived until I was 22, the sky was clear enough to let you observe the planets and the Moon quite well. Maybe that's why I became a planetary scientist.
In the past few months you and the astrophysicist Maud Langlois have been jointly coordinating2 a CNRS programme3 that aims to design instruments to help the scientific community detect evidence of life around stars relatively close to our own. What connection is there between planetary science and the origins of life?
A. M.: We have to assume that life cannot arise anywhere else but on a planet. So, it makes sense to first try to understand how these bodies form. The emergence of life also depends on the characteristics of the planet that harbours it, such as its orbit, how far it is from its star, how it is affected by its moons if it has any, by internal convection in its mantle, by plate tectonics, and by the stability of its climate in the presence of a star that is undergoing rapid change.
The programme I co-direct involves astronomy, geochemistry, geophysics and biology. The biologists are there to tell us what types of environment are likely to be conducive to life. The job of the geophysicists is to understand how such environments can develop on a planet that was initially a world of magma. And we astronomers have to try and figure out how such planets form, how Earth-like bodies and super-Earths come into being, for example. To achieve these goals, we therefore had to come up with new research instruments and design them. I was a little hesitant initially, but you have to face the facts: if we are to unravel the mysteries of the origins of life, we have no choice but to develop new analytical, measuring and detection tools. It was this insight that finally convinced me to fully commit myself to this programme.♦
Find out more on our website
Investigating the origins of life
- 1. J.-L. Lagrange Laboratory (CNRS / Observatoire de la Côte d’Azur / Université Côte d’Azur).
- 2. Centre de Recherche Astrophysique de Lyon (CNRS / École Normale Supérieure de Lyon / Université Claude Bernard Lyon 1).
- 3. Exploratory Priority Research Programme and Infrastructure: Origins (Life and Universe).