You are here
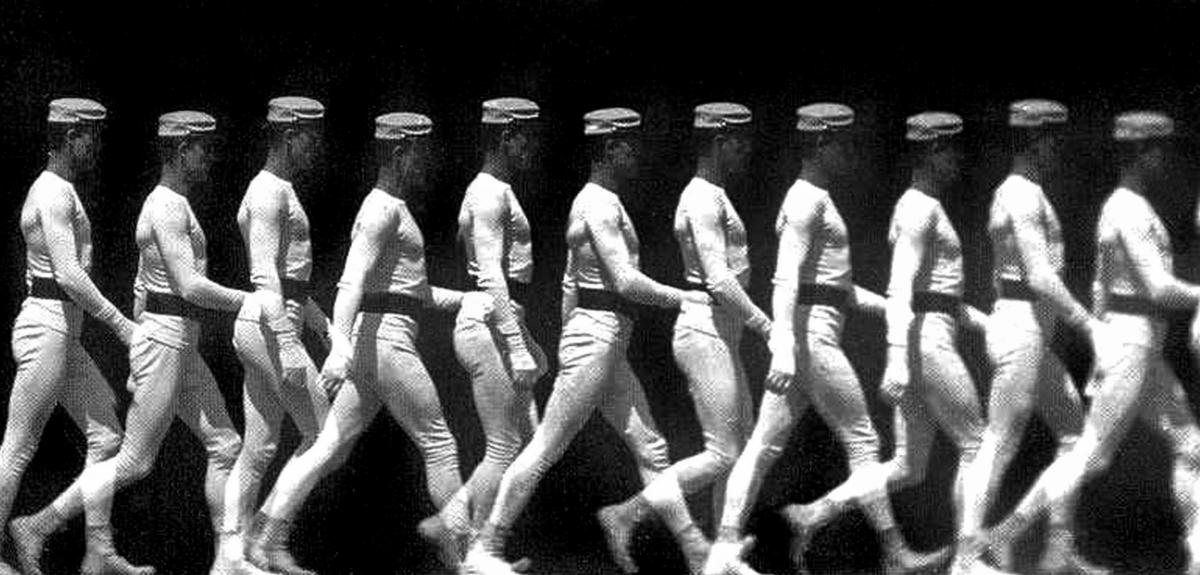
“Take fifteen steps towards the end of the room, then turn around and walk back to where you started.” Easy!—probably a warm-up before moving on to more complicated tasks. You start walking confidently across a floor covered with intelligent tiles, as you would towards the coffee machine, but the assistant is already looking closely at the computer screen. A few return trips later, a glance at the graphs on the printer is enough to understand that this is not exactly a walkover. The length and frequency of steps, the distance between the feet, the force applied by the body on the floor at each movement, the reaction time necessary to turn around… a vast quantity of data has accumulated.
Watching a 3D video of a student on a treadmill, covered with sensors from head to toe, observing the movements of the hips, knees and feet, and even the muscular efforts of the walker transformed into a bionic silhouette leaves no room for doubt. Setting foot into the Aquitaine Institute for Cognitive and Integrative Neuroscience (INCIA)1 shows that taking one step in front of the other is much more complex than it seems!
From neurons to the spinal cord
And this is nothing yet. Two floors up, in the laboratory adjacent to the office occupied by Jean-René Cazalets, head of the Mobility Study Platform, researchers demonstrate that walking is neither a conscious activity driven directly by the brain nor a reflex movement of the muscles in the legs, as was believed until the beginning of the 20th century. In fact it is commanded by an entirely original network of autonomic neurons. Under the microscope, it is indeed possible to observe a fragment of the spinal cord sampled from a very young rat and distinguish the ventral roots—very fine filaments that link the cord to the animal’s muscles. Using a physiological solution (mainly composed of sodium, potassium and calcium) and feeding it with oxygen, this spinal cord fragment can be kept alive for a whole day, explains the scientist. “When you give it a continuous fuel supply, the electrodes placed on the ventral roots record rhythmic impulses, to the right and then to the left, which accelerate gradually until they reach a rat’s speed of locomotion.” In other words, if the muscles were still linked to the spinal cord, they would contract, even though there is no longer any connection with the brain!

While this brings to mind the famous story of the headless chicken that continues to run for a few seconds, Jean-René Cazalets prefers to refer to St Denis of Paris. This Christian martyr, who was beheaded in the 3rd century on the heights of Montmartre, walked slowly down the hill, with his head under his arm, to the future site of the basilica that now bears his name. This image is more striking, as it involves human locomotion, which has raised considerable interest among scientists over the past thirty years.
Already in the early 20th century though, a Scottish physiologist, Thomas Graham Brown, questioned the reflex theory, generally accepted at that time, which postulated that stretching a muscle made it contract. The mechanism is simple: moving the leg forward results in the antagonist muscle (i.e. the muscle that did not make the movement but was stretched) contracting as a reflex and bringing the leg back. The first then reacts in turn to move the limb forward, and so on.
Brown, who was also an experienced climber, felt that this theory was too simplistic. Observing a cat (the "guinea pig" preferred by scientists in those days), he found out that after cutting communication between the spinal cord and the brain and sectioning the dorsal roots responsible for feeding sensory information from the muscles (and also the tendons and skin, etc.) back to the spinal cord, rhythmic and alternating contractions of the flexor and extensor muscles in the ankle (like during walking) persisted for several tens of seconds. And this occurred even though the “reflex information" from the muscle could no longer be transmitted to the spinal cord and brain. In other words, even when deprived of instructions from the brain and peripheral organs (muscles, tendons, skin, etc.), the cat could move its ankle. “This was the first-ever demonstration that the spinal cord contains autonomic neural structures capable of organising movement by themselves,” concludes Jean-René Cazalets.
It was not until the 1990s and the development of new physiological techniques that could sustain the life of an isolated nervous system (once removed, the cord "dies" within several tens of seconds) that it was possible to confirm the presence of this neural network in the spinal cord of mammals, and understand its functioning.
“We have now clearly identified the central pattern generator (CPG), or in other words the mechanism that can produce locomotor activity in the absence of the brain,” explains Frédéric Brocard, senior researcher at the Timone Neuroscience Institute (INT)2 in Marseille (southeastern France). “We also know that this network is located in the lower back and that it generates the right-left alternation of locomotor movements during walking, as well as its rhythm.” As it were, the brain merely commands the start or end of a motion, as often as an obstacle presents itself or a change of direction is necessary.
Once the movement has been initiated, it is the neurons in the spinal cord that trigger basic walking in an autonomous manner. As well as motor neurons, which command muscle contractions, advances in genetic engineering have made it possible to identify the properties of neurons in the locomotor CPG. These so-called “interneurons” are divided into "excitatory" and "inhibitory" subtypes, which ensure the maintenance of left-right alternation. “Another specific feature of interneurons is that they are endowed with independent rhythmic activity, in the same way as that which drives cardiac rhythm in the heart,” adds Frédéric Brocard.
Precise mathematical models
Other more technological advances regarding the capture and analysis of movement have shed fresh light on the mechanisms of walking. The pioneer in this field was a French physiologist, Étienne-Jules Marey. In 1892, he developed chronophotography, a photographic process that breaks down the phases of walking, running or the flight of birds and made it possible, among other things, to understand galloping in horses. Yet biomechanics, which studies the physics of movement, is a very recent discipline. “The term only appeared during the 1970s, and was still somewhat restricted," explains Frédéric Marin, an academic at the Université de Technologie de Compiègne, in northern France. “It is above all the technological and digital advances of the late 20th century that have opened up the possibility of capturing human motion and measuring it with precision.” These advances include digital cameras combined with 3D reconstruction algorithms and inertial units able to detect acceleration and angular speed, such as those that drive the screens of mobile telephones.
This enabled the development of a precise mathematical model of the skeletal and muscular system involved in bipedal walking. A step is broken down into different phases, the main ones being, in sequence, toe-off (when the foot leaves the ground) swing (or the time it is in suspension), heel strike (when the heel comes into contact with the ground) and finally support, when the weight of the body is borne by the leg. Swing, for example, occurs on average two-thirds through the overall movement. Within this timeframe, the model can describe, to the millisecond, the rotation and flexion angles of the joints (hip, knee, ankle, foot), the forces exerted on them and the electrical activity of muscles, etc.
“Because walking has the advantage of being a cyclical, regular, stereotyped and reproducible action, these measurements provide a relatively complete musculoskeletal assessment from which the state of health of an adult can be inferred,” explains Frédéric Marin. There are of course specific parameters—for example those that evolve with age or differences between men and women—, and some grey areas still remain, in particular regarding our understanding of the knee and foot joints, which are more complex than those of the hips. But these models of normal walking are sufficiently accurate to serve as a reference in the context of clinical observations.

An indicator of health status
The therapeutic prospects thus opened largely justify the interest that research laboratories and the medical world have taken in walking. Because it is a complex system that involves the skeleton, muscles and nervous system, walking is a good indicator of an individual’s general health status and can be useful in detecting, treating or monitoring pathologies. “For example, in the case of rehabilitation following an accident or the replacement of knee ligaments, we can follow the course of a patient’s walking parameters,” explains Frédéric Marin. “This is useful from both a purely kinematic approach—which consists in observing movements only—and a dynamic perspective, by looking at the forces that trigger movement.” Measuring support times or their strength, the symmetry or asymmetry of steps (such as when someone is described as having a heavy gait), makes it possible to verify the efficacy of a treatment and also evaluate different types of equipment, such as orthopedic soles.
Just observing a patient’s gait can help to detect neurological disorders at an early stage, such as Alzheimer’s or Parkinson’s diseases which cause locomotor dysfunctions. Identifying and quantifying these dysfunctions, as well as their frequency and extent, can provide hints as to the severity of the condition and its course. A freezing gait (stopping abruptly as if one had lost an automatic ability to walk) is a good indicator of Parkinson’s. Diseases directly related to locomotion can also be explained. This is the case of spastic phenomena, where patients experience very strong and painful muscle contractions that severely hamper their mobility. “For the spinal cord to function normally, a balance between excitation and inhibition is necessary,” explains Frédéric Brocard. “If this equilibrium is disrupted by a lesion, the excitatory interneurons become hyperactive and their inhibitory counterparts much less, thus causing hyperexcitability of the spinal cord that will be transmitted to the muscles via the motor neurons.”
It was long believed that the problem came from the muscles. It is now known that it is linked to the autonomic nervous system within the spinal cord, and even more specifically, that it is caused by the disruption of a small channel carrying sodium to the motor neurons. This could be corrected with a drug used to treat Charcot’s disease, but it is only one of a host of mechanisms that still need identifying.
The mere fact that the CPG, "home" to the interneurons responsible for locomotor rhythm, has been localised already opens the way towards important improvements in the mobility of paraplegics. "In the US, electrodes have been inserted between their L2 vertebra and the spinal column at the level of the CPG. If these are stimulated, movements become more regular than the chaotic twitches normally observed in these patients. By combining this system with training on a treadmill, it appears that it is possible, at least in part, to restore locomotor function, although this is still the subject of debate among scientists, adds Jean-René Cazalets.”
In France, the Cognac-G (Cognition and Action Group)3 neuroscience and neurophysiology laboratory led by Pierre-Paul Vidal works hand in hand with the medical world. It includes clinicians from the Army Health Services and Paris Public Hospitals, as well as CNRS neurophysiologists and engineers, who closely collaborate with the Centre for Mathematical Studies and their Applications (CMLA)4 in Paris-Saclay. “One of our objectives is to collect as much data as we can from all the services we work with, so that we can build the widest possible database on central nervous system diseases and their associated locomotor disorders,” explains Laurent Oudre, one of the group’s mathematicians.
A tablet as a diagnostic aid
In parallel, over the past three years, computer scientists and mathematicians have been designing a user-friendly electronic tablet that physicians can use as a diagnostic aid. The first prototypes, programmed to detect multiple sclerosis, have been available to clinicians for a few months. “All it takes is the tablet, plus wireless sensors and a 10-metre corridor to test the walking skills of the patient,” explains the mathematician. “Algorithms do the rest.” These algorithms had to be encoded when the researchers realised that those used in smartphones to calculate the number of steps or evaluate the performance of joggers, for example, could not be applied in the case of certain pathologies. Indeed, patients who have recently suffered a stroke, or the very elderly, walk in a highly cautious manner; some even drag their feet instead of raising their legs. The standard apps only record the signal emitted by the jolt when the foot touches the ground. “We spent a lot of time with the physicians observing movements of the feet and legs, and this led us to call many things into question when it comes to describing gait,” explains Laurent Oudre.
For the scientists, this work is far from complete, as they have mainly focused on the lower limbs in recent years—and walking does not just involve the legs. “Look at those poor robots who walk so poorly because their torso is limited to a box carried by two legs,” explains Jean-René Cazalets. He filmed his daughter while she was learning to walk: children start by moving one leg forward, but their torso is left behind and may throw them off balance. “Walking is a dynamic posture where both the torso and legs are coordinated.” The scientist therefore decided to look at all the muscles and the spine. “When you ask a subject to walk, you can almost see a wave that propagates from the top of the back and down the vertebrae. This is not a simple, passive mechanical movement but once again the result of the motor programme generated by the interneuron network.”
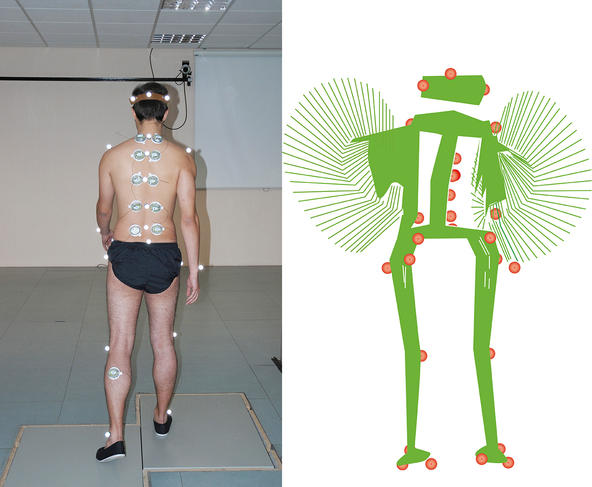
This reminds the researcher of the locomotor system of the lamprey or eel; these fish take support from the water and use their torso to propel themselves forward. Although the torso of biped humans does not serve for propulsion, it continues to participate in the movement. “One could imagine that when hind limbs first appeared, they took control of motor function as a whole,” he suggests, “but nature has not reinvented the entire mechanism.” This hypothesis, which still needs to be confirmed, suggests that walking may take us on the path towards further breakthroughs.
__________________________________________________________________________________
Biomechanics to understand evolution
One question still nags all specialists in the field: why are humans the only animal species to be permanently bipedal? The tools they have developed are now helping their paleoanthropologist colleagues to study the mobility of our distant ancestors, in a bid to answer this broad issue. “The fossilised bones and footprints available to us are too fragmentary to draw any definitive conclusions regarding the mode of locomotion of the early hominids,” explains Gilles Berillon, paleoanthropologist at the HNHP5 laboratory. “Thanks to the modelling tools used by experts in biomechanics and the simulation of movement,6 we are hoping to propose plausible types of locomotion for each species as a function of the configuration of their pelvis or knees, etc.” In order to broaden the reference anatomical model, paleoanthropologists and biomechanics researchers have decided to include, alongside humans, their primate cousins who are occasionally bipedal. This is why baboons equipped with sensors are now walking on treadmills!
The long-term objective is to achieve an optimum reconstitution of the type of bipedalism practiced by Lucy, 3.2 million years ago, Orrorin 3 million years earlier or Neanderthals less than 100,000 years ago. Indeed, contrary to a long-standing belief, the evolution of bipedalism was not linear from chaotic movements to increasingly efficient walking. All hominid species tested different bipedal mechanisms (balance achieved by alternating arm movements, arched feet, knees splayed or not, bent or not, etc.), with varying success. Some morphological aspects of Orrorin’s femur, 6.5 million years ago, are more similar to ours than Lucy’s were, and his ability to walk on two feet is no longer in any doubt. As for the feet of Ardipithecus ramidus, 4.5 million years ago, they already seemed capable of bipedalism and were not simply adapted to climbing or grasping objects.
- 1. CNRS / Université de Bordeaux.
- 2. CNRS /Aix-Marseille Université.
- 3. CNRS / Université Paris-Descartes / Ministère des Armées.
- 4. CNRS / École Normale Supérieure Paris-Saclay.
- 5. Histoire Naturelle de l’Homme Préhistorique (CNRS / Muséum National d’Histoire Naturelle / Université de Perpignan Via Domitia).
- 6. Movement, Sport and Health Sciences laboratory (M2S) in Rennes (northwestern France), and the Laboratory for Analysis and Architecture of Systems (LAAS) in Toulouse (southwestern France).
Comments
Log in, join the CNRS News community