You are here
Mini-organs with maximum potential
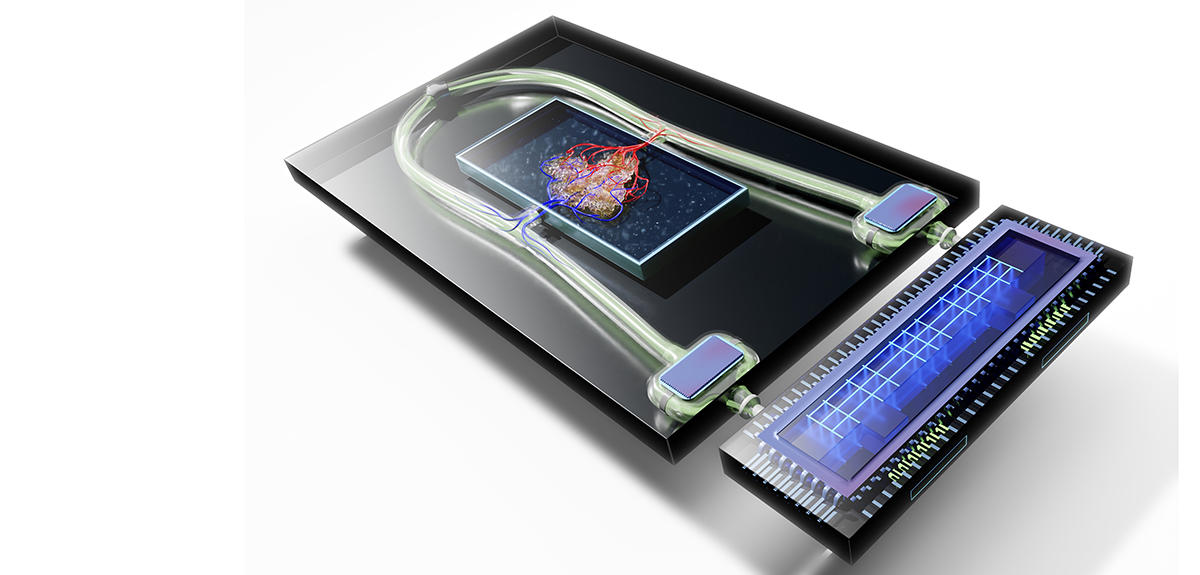
The in vitro growth of miniature copies of the liver, gut, heart, pancreas, kidneys, lungs, prostate, mammary glands or even the brain is not science fiction. Indeed, in recent years, biologists have managed to create all these mini-organs in the laboratory – as well as many others, whose list is steadily lengthening. These organoids (the largest of which measures just a few millimetres) have a three-dimensional structure that resembles that of a whole organ – or part of it – and can reproduce some of its functions. Such accuracy offers fascinating perspectives for research and medicine; using these mini-models, scientists hope to gain a better understanding of the development of organs and the diseases that affect them, to assess the efficacy of new treatments or even to improve the transplantation of cells or organs.
Isolating stem cells
But what’s the recipe for obtaining organoids? The main ingredient is stem cells, which are distinctive for proliferating by division and specialising into any type of cell (neurons, muscle cells, liver cells, etc.). Two types of stem cell are used, the first being those naturally present in certain organs such as the lungs or intestine. “In these organs, stem cells are constantly dividing in order to renew tissues – within a few days in the intestine – and repair them in the event of a lesion. It is this phenomenon that we exploit in the laboratory to produce organoids,” explains Céline Cougoule, at the IPBS.1 The first intestinal organoids were thus created in 2009 by scientists in the Netherlands.
These miniature versions can also be produced from so-called “induced” stem cells (or iPS). These are differentiated adult cells that have been genetically reprogrammed to the state of stem cells. This technique has revolutionised the field of organoid research due to the simplicity of its implementation – adult cells are collected from the skin or blood of an individual – and also because it does not require the controversial use of embryonic stem cells. This is the method applied to obtain retinal or brain organoids (cerebroids).
Cultivating a 3D miniature replica
Once the stem cells have been isolated, they are placed in a nutrient gel that provides them with a matrix to grow under 3D conditions; by adding a cocktail of substances that stimulate differentiation, the cells specialise and organise themselves spontaneously into a three-dimensional architecture that replicates that of a tissue or organ, with the same variety of cell types. This approach, which follows the same processes as those that occur during the formation of an embryo, makes it possible to obtain a sufficiently mature organoid within a week for an intestinoid, or several months for a cerebroid.
Unlike traditional (flat) cell culture on the bottom of a Petri dish, organoids are game-changers. Their 3D structure, combined with the fact that they contain several cell types that can interact in the same way as in a real organ, means that they can mimic its functioning much more successfully. They can also reproduce some of its principal functions; for example, secretion and absorption for an intestinoid and even electrical activity for a cerebroid, as shown by an American team in 2019.
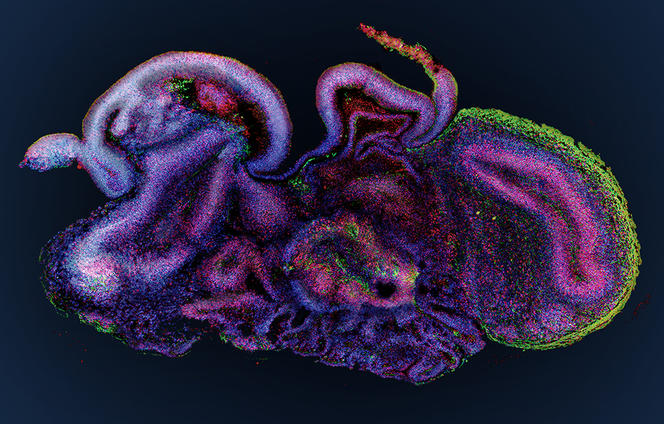
When compared to the animal models used by biologists, organoids represent a breakthrough. “Because they are obtained from human material, they are more predictive of how a disease develops in humans, or how people respond to an infection or drug therapy,” points out Jean-Luc Galzi, directof of the CNRS research infrastructure, ChemBioFrance. It is therefore easy to understand why these miniature replicas, which were initially designed to study the processes at work during the development of our organs – difficult to observe in vivo – now make it possible to envisage numerous therapeutic applications.
Deciphering diseases
First of all, organoids offer excellent living material for deciphering diseases. Indeed, since they can be created using stem cells from patients, these 3D aggregates also reproduce their tissue abnormalities. This enables an understanding of the molecular or cellular mechanisms underlying pathologies. They can also be used to test therapeutic solutions, under the assumption that what will work to “treat” the copy of the organ will have a good chance of also being effective in the patient’s body.
This approach is particularly true with respect to genetic diseases: the cells in organoids carry the same mutations responsible for a disease as those present in the patient and therefore display the same dysfunctions, which can be studied in detail. These advances have already enabled the treatment of patients with cystic fibrosis, a hereditary condition that affects the respiratory and digestive tract and amongst other symptoms causes an excessive production of mucus. The disease is caused by mutations of the CFTR gene that affect a protein found on the surface of cells, reducing the transport of water and salt through their membrane.
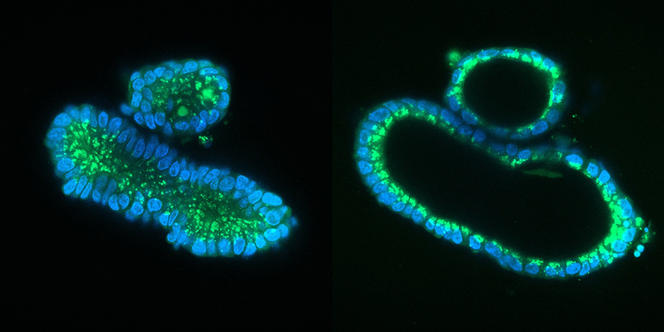
Drugs are now available to improve the functioning of this protein, but in most cases, they are developed and tested in patients with the most common mutations, to the detriment of those carrying rarer mutations. This is where organoids come into play: since 2015, Dutch scientists have been growing copies of intestines derived from cells in the rectal mucosa harvested from these patients. They can thus test the efficacy of drugs on these cell structures by determining whether gene function has been restored. If successful, the molecule can then be prescribed to patients. Céline Cougoule is upbeat: “This personalised medicine, made possible by organoids, is only in its early stages but promises to change the lives of patients in the future”.
Understanding chronic intestinal diseases
Many other pathologies will soon benefit from the contribution of organoids, which are now being used in laboratories throughout the world. In France, where nearly a hundred labs are involved in this new research field, many organs now have their in vitro replicates. At the Digestive Health Research Institute (IRSD) in Toulouse (southwestern France), Nathalie Vergnolle, Audrey Ferrand and their team are developing colon organoids in order to improve their understanding of the cellular processes involved in two chronic intestinal diseases – Crohn’s disease and ulcerative colitis – and in familial adenomatous polyposis, a genetic condition that causes numerous polyps in the colon and can lead to colorectal cancer.
“By comparing healthy and diseased tissue cultures, we have identified the mechanisms involved in the tissue regeneration defect seen in these inflammatory diseases, and observed a link between this pathology and a particular growth factor. The next stage will be to find molecules that can stimulate regeneration in the first case, and inhibit the action of this growth factor in the other, so as to ‘repair’ our organoids. It may then be possible to find treatments for these patients,” explains Audrey Ferrand.
Fighting pulmonary infections
In her work, Céline Cougoule produces lung organoids that are then infected by micro-injection of viruses or bacteria. With her team, she observes how the cells respond to the contamination. In the case of cystic fibrosis – where, because of the accumulation of mucus, the lungs are the focus for the bacterial infections that are the main cause of death among these patients – scientists have shown that in the presence of Mycobacterium abcessus bacteria, organoids from patients displayed high oxidative stress.
“By treating our organoids with antioxidants, we were able to significantly reduce this stress and thus fight the infection.2 In the longer term, this could lead to the development of a treatment to complement antibiotics, to which these bacteria are developing resistance,” the biologist adds. Another important study concerns Covid-19: since the start of the pandemic, the team have been using lung organoids infected by Sars-CoV-2 to test drugs that are already used in other pathologies. Once a molecule has been administered to their mini-organs, the scientists are able to measure its effect on the replication of the virus.
Céline Cougoule was thus able to demonstrate the antiviral and anti-inflammatory action of fluoxetine, which is normally used as an antidepressant, and whose protective effect against Covid had previously been highlighted by a study in patients.3 “Organoids are now becoming essential in preclinical testing and are therefore a means of reducing the use of animal experimentation,” she points out.
Elucidating the causes of neurodegenerative diseases
At the Stem-cell and Brain Research Institute (SBRI) in Lyon (central-eastern France), the group led by Bertrand Pain is cultivating cerebroids. “When compared to animal models, which offer a very poor reflection of the complexity of the human brain, organoids are a model of choice to decipher the mechanisms underlying the onset of cerebral developmental disorders,” notes the scientist. The researchers’ work has already enabled progress in the understanding of a hereditary neurodegenerative disease that destroys the nervous system of children from a very early age: the so-called CACH syndrome, a leukodystrophy that affects myelin, the white substance that coats the nerves.
As well as genetic diseases, cerebral organoids pave the way for numerous advances in the fight against psychiatric diseases (autism, depression, etc.) or neurological disorders (Alzheimer’s, Parkinson’s, etc.). Furthermore, these brain replicates recently helped decipher the mode of action of the Zika virus, which can cause brain growth deficiency, or microcephaly, in foetuses contaminated during the first trimester of pregnancy. And they have also made it possible to test antiviral drugs on infected cerebroids. This approach has been adopted by Bertrand Pain, who in collaboration with virology laboratories, works in particular on encephalopathy (inflammation of the brain) caused by different viruses such as measles.
Olivier Goureau and his team at the Institut de la Vision4 are developing retinal organoids. Their study focuses on retinal dystrophies, neurodegenerative diseases – some of them genetic – that are characterised by a gradual loss of photoreceptors (nerve cells specialised in the reception of light) from the retina and are the leading cause of blindness in industrialised countries (elsewhere, it is more often linked to vitamin A deficiency during childhood). Thanks to their organoids made using iPS cells from patients, the biologists have been able to observe the death of photoreceptors in vitro as it occurs in patients, and to study the mechanisms at play. The scientists are now developing several therapeutic approaches that they are testing on their mini-organs, the initial strategy being to identify molecules that can prevent the death of these cells.
Repairing the retina
Another even more promising avenue is gene therapy. The aim is to either produce a healthy gene via DNA viruses in the cells, or to directly correct DNA mutations, in particular using CRISPR-Cas9, or genetic “scissors”. “We have shown that in our ‘repaired’ organoids, the photoreceptors no longer die. This is an essential proof of concept if we are to develop a treatment for patients one day,” the scientist enthuses.
The last extremely promising solution being explored by the team is cell therapy. The idea in this case is to use organoids as a source of photoreceptors that can then be transplanted into the retina of blind patients and enable them to recover their sight. “At the moment, we have demonstrated this technique in mice: the photoreceptors we transplanted resulted in a partial recovery of sight,”5 explains the biologist. This cell therapy is not only applicable to the retina, it is one of the major applications promised by organoids, whatever the organ.
Targeting cancerous tumours
Another field where mini-organs are raising high hopes is cancer research. Indeed, in the same way as a copy of an organ can be produced, scientists are also able to create the replica of a tumour – or tumoroid – using so-called cancer stem cells, arising from the cancerous tissue of a patient. These tumoroids can thus accurately predict the sensitivity of tumours to the different drugs available, and determine the optimum chemotherapy to be administered to a given patient.
While this costly area of personalised medicine is still reserved for a handful of patients benefiting from a good health insurance scheme, it should become standard in the future. Already, biobanks of tumoroids representative of the diversity observed in patients with different types of cancer (colon, pancreas, prostate, lung, breast, ovaries, etc.) are being built up by laboratories, notably in France. These collections make it possible not only to assess the efficacy of chemotherapies but also to test new cancer drugs.
On the other hand, Sophie Martin from the Laboratoire de Bio-imagerie et Pathologies,6 is developing tumoroids using tissues from patients with head and neck cancers. “For this type of malignancy, the basic treatment using monoclonal antibodies is only effective in 10% to 20% of cases. It is therefore important to predict potential failures so that we can offer patients an alternative,” underlines the scientist. However, until now, no marker predictive of the response of a tumour to this therapy had been demonstrated in this type of cancer. Sophie Martin and her team have now identified such an indicator: a membrane protein present in the tumour cells. “We are currently working on validating this marker and testing new molecules on tumoroids that display resistance to the treatment,” she adds.
Mimicking does not mean replacing
Endowed with innumerable virtues, organoids are facing a bright future. Will they ultimately replace animal models? “There is no doubt that they will considerably reduce animal experimentation by making in vivo testing more targeted. But it will not be possible to do without animals completely if we need to reproduce the functioning of an organism as a whole,” emphasises Bertrand Pain.
Impressive though they may be, organoids are nevertheless only miniature fragments of an organism, and not part of a whole. Furthermore, “these mini-organs are still far from mimicking all the functions of our organs, such as the liver’s detoxification ability or the oxygen exchanges in the lungs”, adds Jean-Luc Galzi. Another limitation is the absence of these structures in the immune, nervous or vascular systems. In the case of vascularisation, the obstacle is non-negligible: without blood vessels to supply them with nutrients and oxygen, the group of cells is restricted in terms of growth and becomes necrotic from the inside. As for immune and nervous cells, they are essential to the functioning of an organ, and in particular its response to a threat.
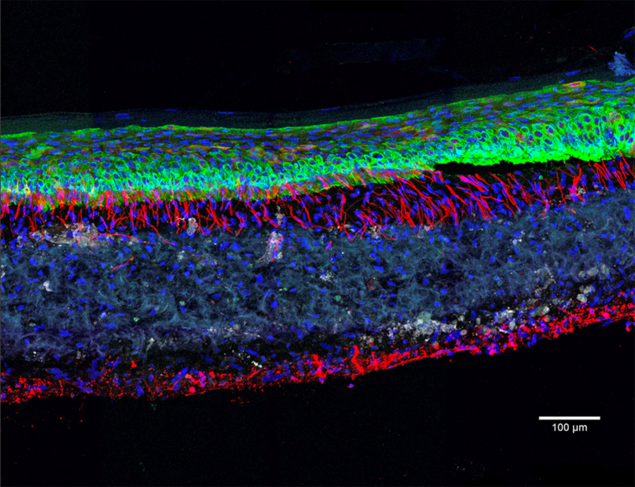
Studying skin healing
Biologists have understood this challenge and are now working on integrating cell types specific to each of these systems in their organoids. This is the case for Vincent Flacher at the I2CT,7 who is developing 3D structures that reproduce the skin with its epidermis and dermis. To achieve this, a collagen matrix is seeded with skin cells to which are added, successively, immune cells (dendritic cells, macrophages), neurons differentiated from iPS cells or endothelial cells whose network imitates vascularisation. “Using these realistic skin models, we can study the interactions between the immune and nervous systems, which play a key role in healing, in inflammatory diseases such as psoriasis or atopic dermatitis, and also in viral infections (Zika, dengue, Chikungunya). We can then try to identify molecules that are effective against them,” explains the biologist.
Implanting a replacement organ
Another way to enhance realism is to implant organoids on chips, microfluidic systems that are able to “perfuse” the mini-organs via microscopic channels and to measure their activity in real time. Using such systems, it is now possible to connect different organoids together in order to study their interactions. In France, a project that relies on this method to combine liver and kidney, or lung and heart organoids is now under way to assess the toxicity of pharmacological molecules, an essential step in the marketing of any new drug. Other teams are already imagining the development of an actual body on a chip. In the longer term, all these amazing advances may help make one of the extraordinary promises offered by organoids come true: beyond the simple cell therapy, the possibility of reproducing a functional tissue or organ in vitro so that it can be grafted in a patient.
Meanwhile, these mini-organs raise numerous questions. Some people believe that the creation of increasingly complex cerebroids resulting from the fusion of organoids from different regions of the brain may lead to the emergence of a form of consciousness, whether this is intentional or not. They consider that it is therefore necessary to bear in mind that these sophisticated entities may be capable of “feeling”, in which case ethical rules should be applied to them (see insert below).
Furthermore, “although organoids offer new perspectives in the field of regenerative medicine, we need first of all to measure their impact on patients. Because these synthetic organs are grown outside the body, without natural interactions with other organs, they will necessarily have different properties. In addition, there is a risk they may develop tumours over time”, warns Jean-Luc Galzi. Between promises, fantasies and risks, we haven’t heard the last of organoids.
________________________________________________
Unprecedented ethical questions
Because they are not natural entities but replicas of our organs created by biological engineering from stem cells, organoids raise some unprecedented ethical questions. Are they simple objects? Or should they be given the moral status of the entity they are mimicking? With respect to “simple” organoids (liver, kidney, intestine, etc.), the answer is clear: like any other natural human biological material collected by a research centre, the ethical questions they pose require informed consent being obtained from the donors of the cells necessary for their production, and relate to the safety, use or even commercialisation of these organoids.
On the other hand, other more “sophisticated” organoids deserve serious moral consideration. First of all, there are what biologists call embryonic models for scientific use, or organoids that recapitulate the initial stages of embryonic development. These include blastoids, which are extremely similar to an embryo; indeed, embryonic appendages – the organs contained in a fertilised egg, external to the embryo and that nourish and protect it – are present. In the mouse, experiments have led to the birth of pups from blastoids implanted in the uterus of a rodent.
Although such experiments are unthinkable in humans, “the extent to which these organoids mimic an embryo is such that safeguards must surround their production. Biologists could thus be required to obtain an authorisation to be able to conduct research on blastoids,” notes Hervé Chneiweiss, Chair of the Ethics Committee at the French national health and medical research institute INSERM, and director of the IBPS Paris-Seine Neuroscience department.8 The same applies to brain organoids (cerebroids). The advances achieved in recent years suggest that it may be possible in the future to create complex cerebroids that reproduce the complete structure of our brain and its different regions. Scientists will therefore have to ask themselves whether such entities might feel pain, or even develop a form of consciousness.
Ethical experts have already proposed that scientists should act “as if” and apply the ethical rules inspired by animal experimentation to such complex cerebroids: these models should only be used if the knowledge they might potentially generate is worth the suffering that could be inflicted, and which should be minimised; for example by administering anaesthetics. “This prospect is still fictional as current cerebroids are far from rivalling the complexity of our brains, provided that such cognitive activity might even exist separate from a body. However, this question must be taken into account; work on cerebroids will need to be better regulated in the future and methods developed to detect their capacity for feeling. Only in this way will research on organoids bear fruit, the general public be confident and useless fantasies be avoided,” concludes Hervé Chneiweiss.
- 1. Institut de Pharmacologie et Biologie Structurale (CNRS / Université Toulouse III - Paul Sabatier).
- 2. “Cystic fibrosis patient-derived bronchial organoids unveil druggable pathways against Mycobacterium abscessus infection”, doi: https://doi.org/10.1101/2022.01.03.474765.
- 3. “Association entre l’utilisation d’antidépresseurs et la réduction du risque d’intubation ou de décès chez les patients hospitalisés atteints de COVID-19: résultats d’une étude observationnelle”, Nicolas Hoertel et al., Psychiatrie Moléculaire, n° 26, February 2021.
- 4. CNRS / INSERM / Sorbonne Université.
- 5. “Restoration of visual function by transplantation of optogenetically engineered photoreceptors”, Marcela Garita-Hernandez et al., Nat. Commun., October 2019; 10(1):4524.
- 6. Unité CNRS/Université de Strasbourg.
- 7. Laboratoire Immunologie, Immunopathologie et Chimie Thérapeutique (CNRS / Université de Strasbourg).
- 8. CNRS / INSERM / Sorbonne Université.