You are here
Friendly bacteria
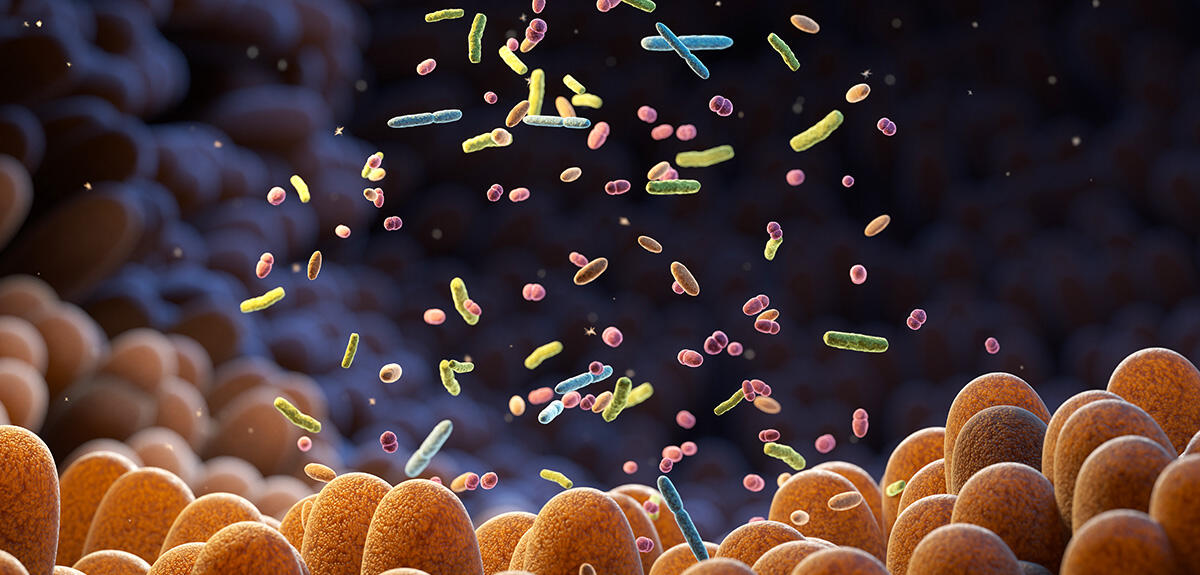
From the hydrothermal vents that pepper the ocean floors and the permafrost of Alaska or Siberia to our own digestive system, no environment can escape colonisation by bacteria. Indeed, despite their microscopic size, these organisms weigh heavily in the balance of life. American scientists have calculated that all those found on Earth represent a total mass of 70 billion tonnes of carbon1 scientists prefer this unit of measurement because it is independent of the water content in living beings, which varies considerably from one species to another, Ed. note. After plants, bacteria thus constitute the second largest life form in terms of biomass.
While these single-cell organisms with exceptional capacities for adaptation have always intrigued microbiologists, they are now arousing the curiosity of chemists who are seeking to develop new medicines. Victor Duarte in the Chemistry and Biology of Metals Laboratory (LCBM)2 in Grenoble (southeastern France), is one of these pioneers. Working with other scientists at the Institute of Molecular Sciences of Marseille (ISM2)3 and the IMM,4 this biochemist has made our intestinal microbiota one of his preferred fields of investigation.
A thousand times more effective than an antibiotic
“Among the hundreds of billions of microorganisms that live in our digestive tract, some produce substances with antibiotic properties. This is the case of the bacterium Ruminococcus gnavus, in which we have identified five different antimicrobial molecules, including Ruminococcin C1 (RumC1)”, explains the LCBM scientist. For several years, this peptide has been the subject of intense study by Duarte and his colleagues. After managing to synthesise it in the laboratory, they sought to demonstrate its antibacterial potential. The team thus observed that RumC1 was active at low doses on several bacteria involved in nosocomial and intestinal infections.5
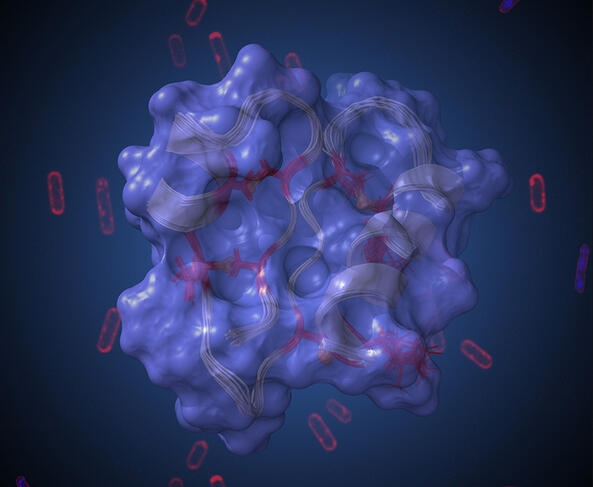
Although the mode of action of RumC1 remains mysterious to some extent, the scientists know that it differs from that of the most widely used antibiotics. “While molecules in this family neutralise pathogenic microorganisms by punching holes in their membrane, our peptide penetrates into the bacterial cell and disrupts one or more biological synthetic pathways such as those for DNA or proteins,” the researcher explains. Many of the characteristics of RumC1 point to its possible therapeutic use.
First of all, its highly compact structure endows it with marked resistance to physiological conditions and to a variety of physical and chemical treatments. Furthermore, the peptide does not display any toxicity towards intestinal cells. Its efficacy has also been demonstrated in mice infected by a bacterium that triggers a form of peritonitis. “The RumC1 dose that enabled elimination of the pathogen from the digestive system of rodents proved to be a thousand times lower than that of an antibiotic widely employed to treat this type of infection,” Duarte enthuses.
At a time when the World Health Organization (WHO) is predicting that antibiotic resistance will be responsible for 10 million deaths a year by 2050, RumC1 offers a promising alternative to current antibiotics whose efficacy is constantly declining.
A protocell based on bacteria
Being able to mimic all the reactions that occur in a living cell is the quest of many chemists and biologists. Although several scientific teams have already managed to reproduce certain essential cell functions, such as enzymatic cascades or the synthesis of RNA and proteins from DNA, integrating them within the same compartment has proved a much more arduous task. Scientists at the University of Bristol (UK), working in collaboration with a chemist at the Paul Pascal Research Center (CRPP)6 in Bordeaux (southwestern France) have nevertheless managed to take up this challenge by integrating microorganisms in polymer microdroplets.7 “We just applied the principles of the famous German physician Rudolf Virchow 1821-1902, Ed. note according to which ‘all cells come from pre-existing cells’”, summarises Nicolas Martin, a CNRS researcher at the CRPP.


The so-called “bottom-up” strategy adopted to construct this artificial cell is nevertheless groundbreaking. It consists in extracting from living organisms – in this case, Escherichia coli (E. coli) and Pseudomonas aeruginosa – the building bricks that enable any cellular unit to function. Once imprisoned in the microdroplet, which acts as a cytoplasm, the bacteria are destroyed using chemical products so that they can release their cellular components (referred to as cell lysis), such as DNA, proteins or lipids, etc.
In order to produce an ersatz nucleus, the bacterial DNA is then concentrated within the microdroplet by adding histones, basic proteins associated with chromosomal DNA. At that stage, it only remains to ensure the energy autonomy of this protocell, for which E. coli are once again called upon. Implanted after the cell lysis process so as to keep them alive, these bacteria act as mitochondria. By inducing the formation of a protein cytoskeleton within their protocell, the scientists have even managed to make it adopt morphological features similar to those of a eukaryote cell.
Although this research is still in its infancy, it promises potential applications in the health field. “Such artificial cells could be used to replace failing ones in certain organs, or to synthesise medicines,” suggests Martin.
A microorganism with magnetic powers
As their name suggests, magnetotactic bacteria use the terrestrial magnetic field to position and move themselves. This ability results from the presence of intracellular organelles that behave like magnets: magnetosomes. A consortium of scientists from several French laboratories8 took inspiration from this phenomenon to design a magnetic bacterium. The first stage in this work9 consisted in genetically modifying E. coli bacteria so that they would produce ferritin. This protein, which resembles a cage of nanometric dimensions, enables the storage of iron within the bacterium. In a second phase, iron in the form of metallic salts was added to the culture medium for the bacteria.
“After being captured by the microorganism, the metal oxidised to form a nanoparticle which endowed it with magnetic properties, while at the same time protecting it from an excess of iron that would be fatal,” explains Zoher Gueroui, CNRS research professor at the Laboratoire Pasteur10 in Paris, who led these investigations. Once modified, the microorganism can be controlled very easily using simple magnets. Scientists have already explored the potential applications of these magnetic bacteria, including their possible use as biosensors.
The principle, which has been the subject of a patent filing, is as follows: in response to the biochemical signature of a pathogenic agent, the magnetic bacteria emit a fluorescent signal. “By applying a magnetic field, it is then possible to concentrate the light signal emanating from each bacterium and thus markedly enhance the sensitivity of the measurement,” Gueroui adds. By offering an opportunity to detect an infection at an early stage, this method could, for example, aid in the early diagnosis of infectious diseases.

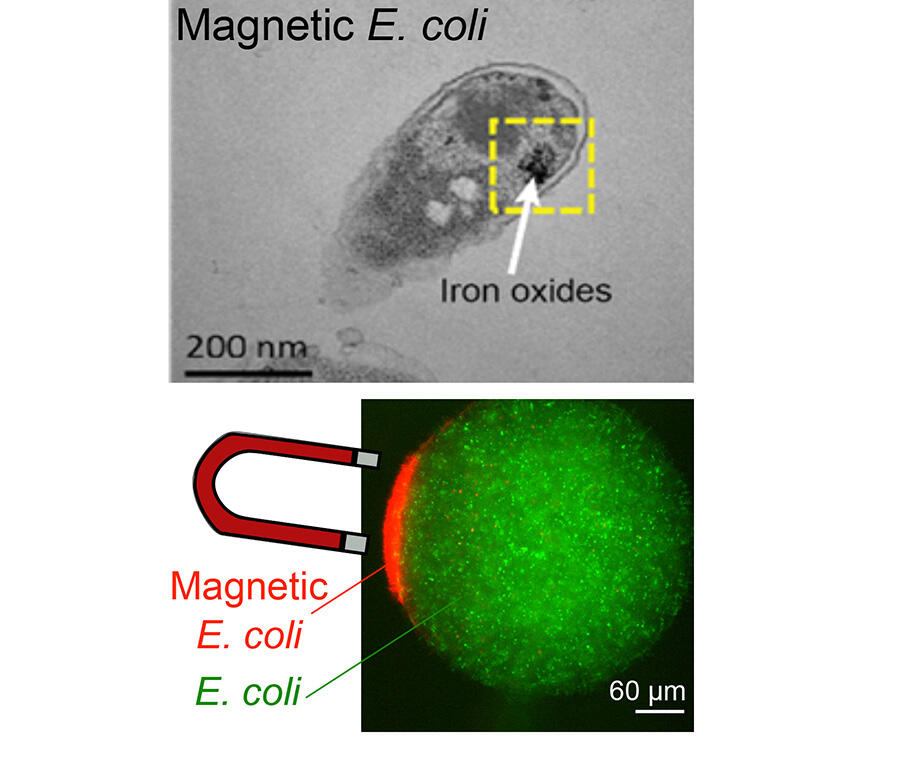
Research on bacteria raises hopes of numerous other innovations in the field of biosensors. One of these could revolutionise the analysis of hormone levels. Resulting from a Franco-American collaboration, including scientists from the LCPO,11 this work has enabled the design of a progesterone biosensor using bacterial transcription factors. Unlike current hormonal biosensors which cannot measure fluctuations in the concentrations of these substances, this solution would offer continuous readings of progesterone levels.
By modifying the molecular structure of a receptor used by a bacterium to bind to human cells, a Franco-Austrian team12 has succeeded in rendering it specific to certain blood groups. This first step towards the development of programmable biosensors could pave the way for a broad range of applications in medical diagnostics.
- 1. “The biomass distribution on Earth”, Yinon M. Bar-On et al., PNAS, May 2018. https://doi.org/10.1073/pnas.1711842115(link is external).
- 2. CNRS / CEA / Université Grenoble Alpes.
- 3. CNRS / Centrale Méditerranée / Aix-Marseille Université.
- 4. Institut de Microbiologie de la Méditerranée (CNRS / Aix-Marseille Université research federation).
- 5. “The unusual structure of Ruminococcin C1 antimicrobial peptide confers clinical properties”, Clarisse Roblin et al., PNAS, July 2020. https://doi.org/10.1073/pnas.2004045117(link is external)
- 6. CNRS / Université de Bordeaux.
- 7. “Living material assembly of bacteriogenic protocells”, Can Xu et al., Nature, September 2022.
- 8. Laboratoire Pasteur (CNRS / ENS-PSL / Sorbonne Université), Institute of Mineralogy, Physics of Materials and Cosmochemistry (IMPMC – CNRS / MNHN / Sorbonne Université), Institute of Biology of the Ecole Normale Supérieure (IBENS – CNRS / ENS-PSL / Inserm), CIRB (CNRS / Collège de France / Inserm).
- 9. “Engineering E. coli for magnetic control and the spatial localization of functions”, Mary Aubry et al., ACS Synthetic Biology, September 2020.
- 10. Processus d’activation sélectif par transfert d’énergie uni-électronique ou radiatif (CNRS / ENS-PSL / Sorbonne Université).
- 11. Laboratoire de Chimie des Polymères Organiques (CNRS / Bordeaux INP / Université de Bordeaux).
- 12. CERMAV (CNRS) and Austrian Centre of Industrial Biotechnology (ACIB).
Author
After first studying biology, Grégory Fléchet graduated with a master of science journalism. His areas of interest include ecology, the environment and health. From Saint-Etienne, he moved to Paris in 2007, where he now works as a freelance journalist.