You are here
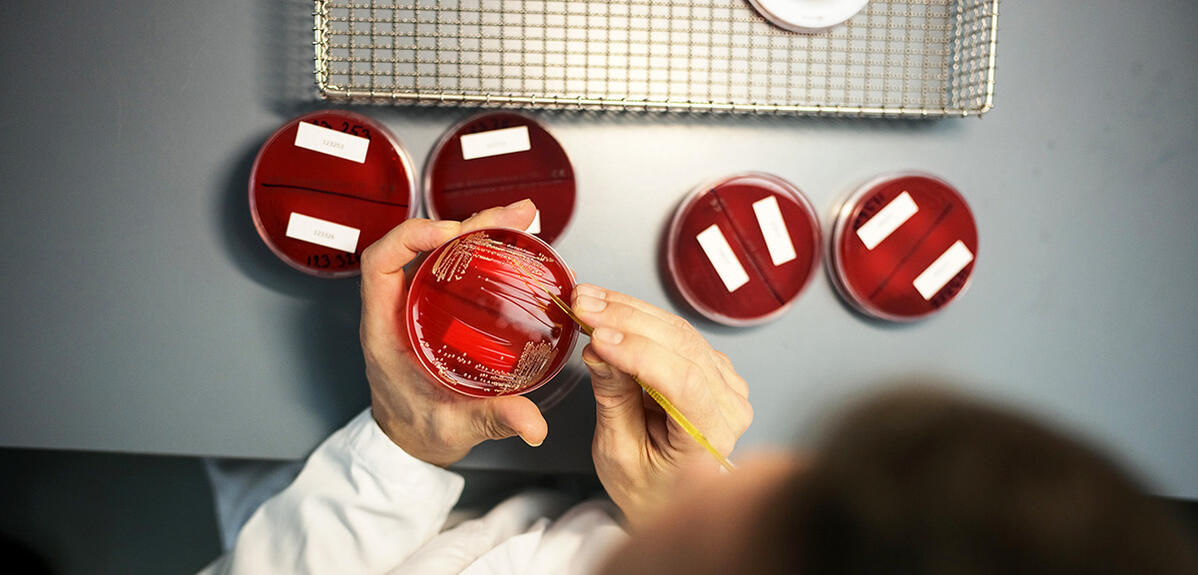
There is consensus that if nothing is done, the resistance of pathogens to antimicrobial agents could become the leading cause of deaths worldwide by 2050. As confirmed by the World Health Organization (WHO) as early as 2015, this phenomenon “represents an immense danger to global health” that could lead to a “post-antibiotic era in which common infections and minor injuries can kill”. This would be like returning to the pre-penicillin era… Aware of this danger, scientists throughout the world are now tackling the problem. Biologists and chemists are in the front line, along with experts from the CNRS and its partners.
The acquisition of resistance to antimicrobials, the medicines used to prevent and treat infections, is a natural process. Pathogens evolve over time, with natural selection favouring the micro-organisms best suited to their environment, in this case rendering them insensitive to the drugs to which they are exposed. Only in recent decades, the sometimes inappropriate and excessive use of antibiotics has meant that this phenomenon is reaching a turning point, placing us in a critical situation.
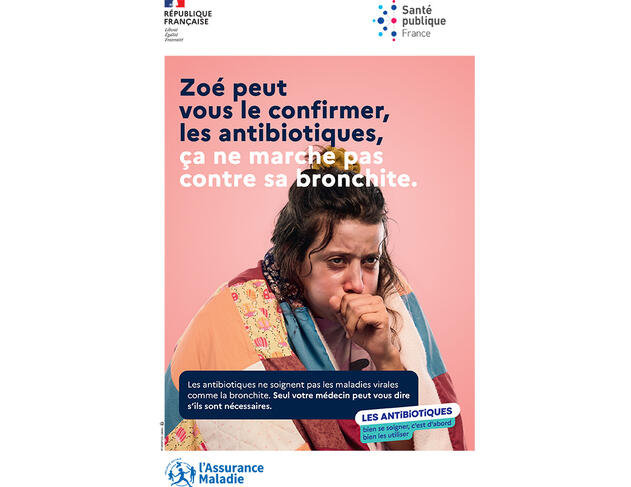
In practice, we are now seeing high rates of resistance amongst common bacterial infections. Because of the rapid global spread of so-called “multi-resistant” pathogens, some of them can indeed no longer be treated using existing drugs. For the past century or so, the development of antibiotics had led us to think that risks of infection were under control, but in this emergency situation we now need to learn again that the fight against infections must be unceasing. This implies a detailed understanding of the processes by which a microbe acquires resistance and how this spreads, and requires constant innovation in terms of the design of diagnostics, antimicrobial agents and the definition of therapeutic strategies.
Understanding the different causes of resistance
As Olivier Neyrolles, at the IPBS pharmacology and structural biology institute1 in Toulouse (southwestern France) explains, “there may be several causes for the resistance of a pathogen to an antimicrobial. It may be linked to a mutation in the target, which is no longer recognised by the drug, or to a change to an enzyme that is necessary to activate this agent. In some cases, it is the membrane of the pathogen that has become impermeable to drugs, or the pathogen has become increasingly able to excrete them”.
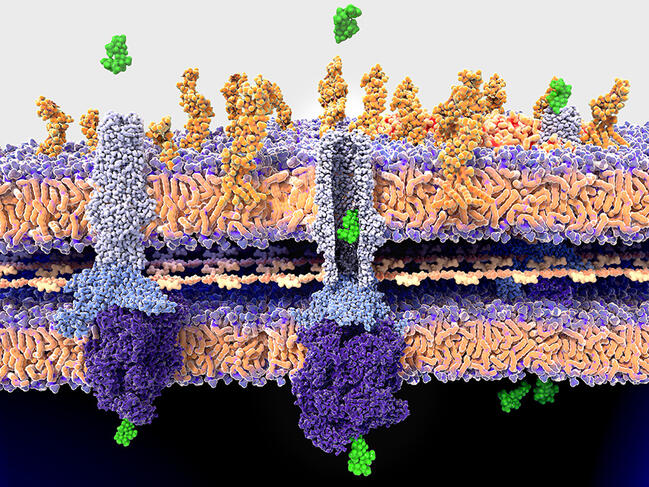
The first mission of specialists is therefore to understand in as much detail as possible the different pathways adopted by resistance, and how the causal genetic mutations occur and spread. For example, Philip Supply, at the Centre of Infection and Immunity of Lille,2 recently determined the factors that allowed the emergence and rapid spread of the so-called W148 epidemic branch of tuberculosis strains. Particularly virulent, W148 strains are also resistant to up to eleven drugs. The result is that not only does this variant of the pathogen display numerous resistance mutations, but also mutations that can compensate for the metabolic cost of their acquisition and a hypervirulence mutation. “Our data has shown that the latter existed before the resistance mutations. So it is the combination of all of them that makes this variant so dangerous,” explains the scientist.

This is notably the case for Acinetobacter baumannii, a bacterium causing opportunistic and nosocomial infections that are difficult to treat, and which is classified by the WHO as a high priority for study and the discovery of new antibiotics. Last year, Xavier Charpentier, at the CIRI international research centre for infectious diseases,3 demonstrated that strains of this bacterium, in principle susceptible to an antibiotic, were able to acquire resistance within a few hours. They achieved this by capturing and incorporating part of the genome of their neighbours. “We still do not fully understand the conditions that govern this transfer, but we saw that up to 80 new genes could be acquired by a bacterium during a single transfer event, which suggests a formidably efficient process,” he explains.
Adapting treatments
“The goal for the scientific community is now to produce a global map of resistance through genomic, expression, proteomic and metabolomic analyses,” comments Paola Arimondo, who leads the Epigenetic Chemical Biology team at the Chemistry for Life Sciences (Chem4Life) laboratory.4 “This will enable us to anticipate any resistances that occur so that we can adapt treatments as a function of the type of resistance we are facing, whether this be at the scale of a patient or at the national level, and we shall also be able to regulate the use of antibiotics in human, animal and agricultural health,” adds the biologist.
In this context, Supply, in collaboration with the company GenoScreen, has developed new molecular diagnostic tools for leprosy and tuberculosis. Based on the technique of deep targeted sequencing, they can use a sample from a patient – which does not need to be cultured – and determine the resistance profile of the strain present so that treatment can be optimally adapted. “In the case of tuberculosis, our test – which is the most wide-ranging at present – can simultaneously establish the susceptibility or resistance of a strain to 13 classes of antibiotics,” the scientist points out. After a patent was filed in 2018, a licensing contract was signed with GenoScreen in 2021 to exploit the discovery. This Lille-based company, in northern France, has just announced the signature of a partnership with Illumina, the global leader in sequencing.
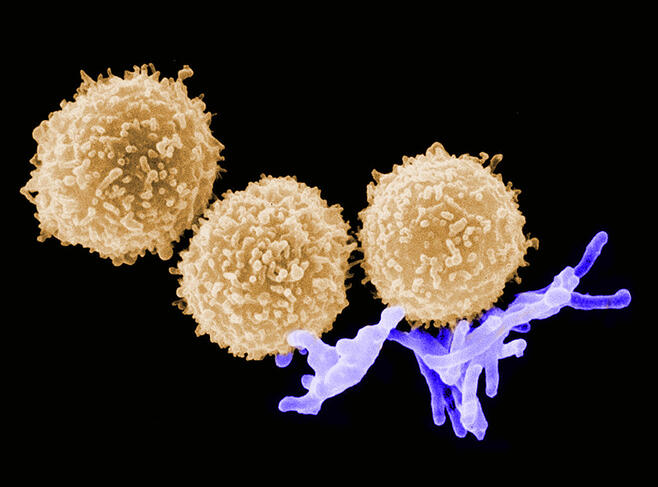
As well as our ability to diagnose and monitor the evolution of mutations, the question of treating infections remains crucial. Indeed, faced with the waning efficacy of antibiotics, all our treatment regimens need to be reviewed by modifying the antibiotic cocktails currently in use and designing new drugs. This challenge has been taken up by Jean-François Hernandez, at the IBMM Max Mousseron biomolecules institute5 in Montpellier (southern France). This chemist is precisely working on molecules that could counteract certain modes of resistance among so-called Gram-negative bacteria to antibiotics in the carbapenem family. These are only used in hospital as a last resort, and the issue of resistance to their effects has been classified as an ultra-priority by the WHO.
Counteracting the adaptation mechanisms of pathogens
Among the numerous adaptations made by these pathogens, one is based on the expression of a family of enzymes called metallo-beta-lactamases, which are able to inactivate carbapenems. This family emerged some thirty years ago and has since become widespread, without an inhibitor of these enzymes being available on the market. After a fortuitous observation made in 2006 by Otto Dideberg’s team at the IPBS, followed by patient work to achieve optimisation, Hernandez and his colleagues managed to develop a series of molecules that could inhibit the action of these metallo-beta-lactamases. A patent was filed in 2018, and a pre-maturation programme was funded by the Technology Transfer company AxLR. “Both in vitro and in vivo, we were able to show that our molecules could restore the effects of a carbapenem on certain particularly problematic clinical strains producing these enzymes,” explains the researcher. “This is very promising and we are now looking for partners so we can go further.”

Among the different strategies currently under study, one consists in trying to target “non-essential” processes of a pathogen. As Neyrolles puts it, “what we mean is processes which, if inhibited in vitro, do not prevent the pathogen from proliferating, but which in vivo are crucial at a specific point in its life cycle”. The interesting point is that because they are less vital to the pathogenic agent, they are less subject to selection pressure. Their targeting should not therefore give rise to the very rapid onset of resistance.
At the IPBS in Toulouse, several teams are working in this direction in order to propose new treatments against tuberculosis. The WHO estimates that nearly half a million new cases of the disease each year prove to be resistant to the main, first-line antibiotics used for treatment. Thus one of these teams has identified an enzyme which is involved in the synthesis of lipids in the bacillus envelope. “When it is deprived of this enzyme in vitro, the bacillus is not affected. However it is damaged if targeted at a particular stage in the infectious cycle”, says Neyrolles.
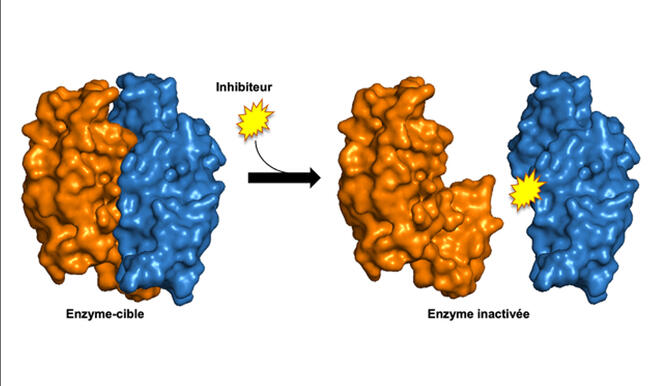
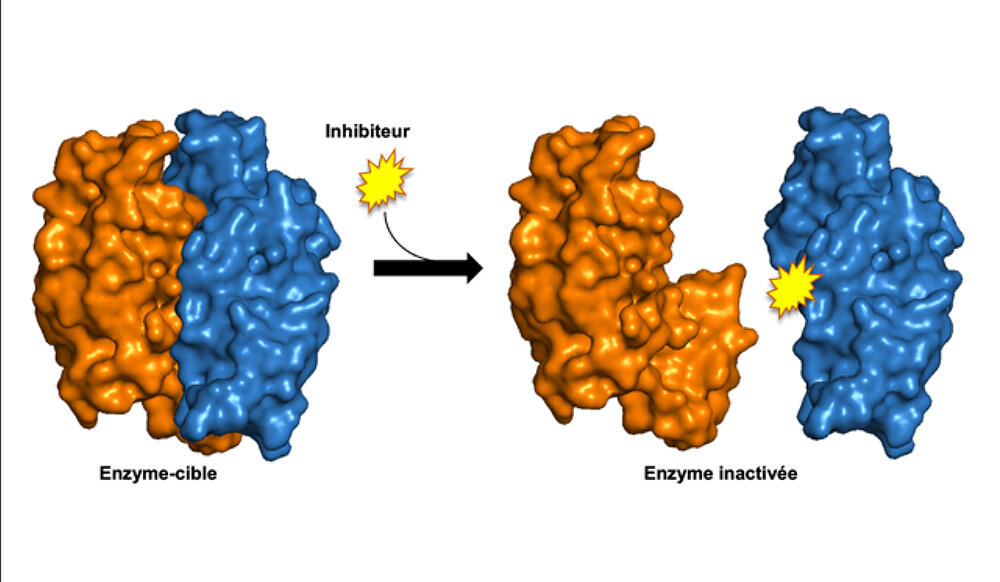
Following this observation, the scientists identified and validated compounds that could destabilise this enzymatic complex. A patent was filed in 2022 and in vivo tests are now planned in order to improve certain aspects of the pharmacokinetics. In parallel, another team in the laboratory recently initiated a programme to find molecules that could attack the PMT enzyme which is also involved in the infectious cycle of tuberculosis. Finally, and much further upstream, a third team is trying to destabilise a toxin/antitoxin system specific to the bacillus in the hope that it could be made to self-destruct.
Focusing on epigenetic processes
On their side, scientists in the Chem4Life laboratory are focusing on the possibility to exploit epigenetic processes in the fight against resistant infections. As Arimondo explains, “epigenetics concerns reversible chemical changes to DNA and the proteins around which DNA is wrapped, which means that in cells of different types, or in different environments, the same genome is not expressed in the same way”. Thus at different times in its infectious cycle, a pathogen will undergo epigenetic modifications to its DNA so that it can adapt its functioning to the different environments it encounters (animal reservoir, different host cells, etc.). Hence the idea of focusing on the proteins involved in these modifications in order to disrupt the life cycle of an infectious agent.
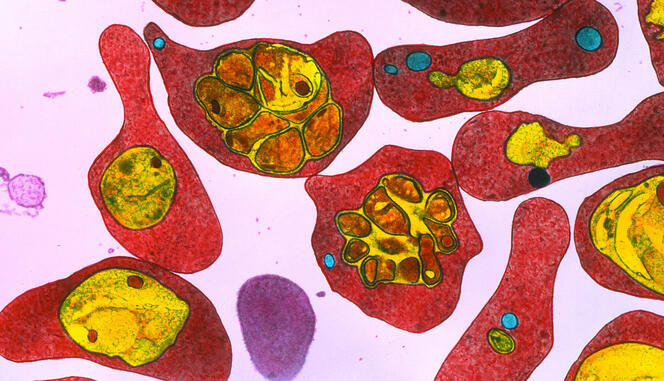
In practice, in 2018, the scientist’s team and that led by Artur Scherf in the Biology of Host-parasite Interactions6 at Institut Pasteur, discovered several series of molecules that could inhibit the proteins associated with epigenetic modifications of malaria. “Tests showed that these molecules are active on some fifty resistant strains,” stresses Arimondo. “We filed a patent in 2021 and are now looking for industrial partners so that we can advance our research.”
In the longer term, and as part of a project involving eight research teams, these scientists are also planning to target proteins in seven bacteria whose role is to modify the epigenome of the host in order to proliferate and grow; for example by escaping from the immune response of the contaminated organism. As a complement to more standard antimicrobials, this approach could also offer solutions to control resistance.
Using microbes to overcome other microbes
One thing is certain; in 2020 the WHO deplored the lack of clinical development of new antimicrobial agents, and now all avenues deserve to be explored. One of them, taken by Véronique Éparvier at the ICSN natural substances chemistry institute,7 consists in looking for solutions, including those directly offered by living organisms. A specialist in the symbioses between micro-organisms and plants or animals, this chemist was behind the creation of the CNRS strain library. This collection contains around 2000 microbial strains that offer an inexhaustible source of tens of thousands of molecules, some of which exert antibiotic effects that could be exploited for use in human medicine.

In the context of collaborations with the Centre National de Résistance aux Antibiotiques, Véronique Éparvier isolated antimicrobial molecules produced by fungi that are symbiotic with termites. She explains: “Our initial tests showed that these molecules were active against fungi in the trichophyton genus which causes dermatophytosis (ringworm) and also against methicillin-resistant Staphylococcus aureus.” Furthermore – although not yet tested on human pathogens – she has isolated cyclic peptides synthesised by endophytes of a palm species that are active against certain plant pathogens.
Yet Arimondo remains cautious: “it should not be thought that we have found a solution. We are talking about long-term studies that will require a great deal of work and investment.” Supply is more optimistic: “I think that institutions and academia have now become fully aware of the problem.” An encouraging assessment, since antibiotic resistance is a certainty, and the clock is ticking.
- 1. CNRS / Université Toulouse III - Paul Sabatier.
- 2. CNRS / CHU de Lille / Inserm / Institut Pasteur de Lille / Université de Lille.
- 3. CNRS / École Normale Supérieure de Lyon / INSERM / Université Claude Bernard – Lyon 1).
- 4. CNRS / Institut Pasteur.
- 5. CNRS / École Nationale Supérieure de Chimie de Montpellier / Université de Montpellier.
- 6. Genetic and Molecular Basis of Host-parasite Interactions Joint Research Unit (CNRS / Inserm / Institut Pasteur).
- 7. CNRS / Université Paris-Saclay.
Keywords
Share this article
Author
Born in 1974, Mathieu Grousson is a scientific journalist based in France. He graduated the journalism school ESJ Lille and holds a PhD in physics.
Comments
Log in, join the CNRS News community