You are here
Focusing on targeted treatments against cancer
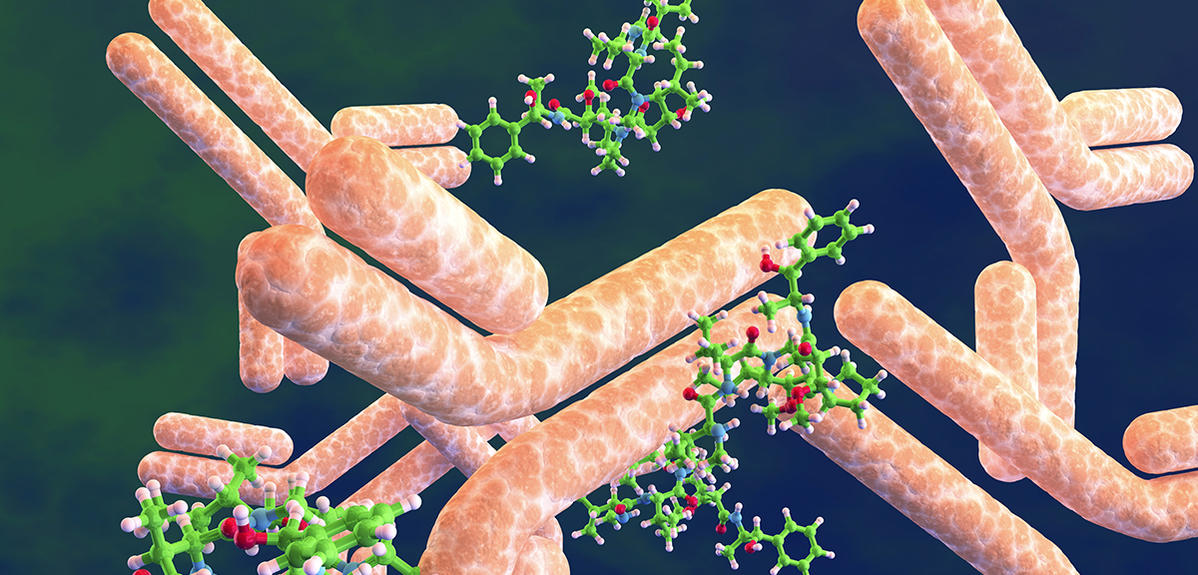
Strike at the enemy’s heart, forcefully and without collateral damage; that is the credo of targeted cancer therapies. Their principle is the same as that of the wooden horse used by the Greeks to enter the city of Troy: a cytotoxic agent carried by a vector that can blend into the bloodstream and deliver its drug load on reaching its destination within tumour cells or in their nearby environment. The idea behind these therapies dates back from the 1980s. It started to bear fruit during the past ten years, and a small number of drugs operating under this principle are now available. Many others are being developed, notably in laboratories run by the CNRS and its partners, with – as a bonus – the creation of several start-ups and the hope of widening the range of weapons used to fight a disease that kills 160,000 people in France each year.
First vectorisation strategy: antibody-drug conjugates
The most advanced vectorisation strategy to date is that of antibody-drug conjugates (ADCs), which consists in binding a cytotoxic molecule to a large biological molecule – a monoclonal antibody – that can fix specifically on a protein site in cancer cells. Once the coupling has reached its destination, the chemical bond between the antibody and the drug is broken down (for example by enzymes present in the tumour environment) thus enabling the latter to exert its toxic activity.
The first treatment based on a conjugate was designed to target lymphomas and was authorised in 2011; today, around a dozen are available. “We have passed a milestone in recent years and are now seeing the accelerated implementation of ADC therapies,” explains Mouad Alami, a scientist in the BioCIS laboratory.1 In practice, tumour biochemistry, medicinal chemistry, the ability of vectors to efficiently reach their targets, and control of the chemical bonds between antibodies and drugs are all factors underpinning the development of conjugates, which requires considerable efforts in research and innovation. This is particularly true because each tumour has its own characteristics, and the issue of adverse effects remains crucial; furthermore, account must always be taken of the uncertainties and random events that may occur during scaling-up from the laboratory bench to clinical trials. Many options are therefore being explored, but notable advances are few and far between.
More stable conjugates that last in the body
For example, in the Laboratory of Design and Application of Bioactive Molecules,2 Alain Wagner and his team have become specialised in precision chemistry that enables the binding of an active ingredient to its “carrier”. “The challenge is to be able to control how many drug molecules we can attach to an antibody, how we can do that, and how these ais are released”, summarises the chemist. Thus, in 2013, the team managed to develop a protocol that ensured the durability of a conjugate over time. Sasha Koniev, then a doctoral student in the laboratory and now director of the start-up Syndivia, set up in 2014 to exploit this discovery, explains: “conventional chemical bio-conjugation processes produce bindings whose stability is not long-lasting. And the problem when treating solid tumours – which are more difficult to reach – is that the conjugate needs to remain in the bloodstream for several days to be effective”. Called APN linker, this process was patented in 2014. Working in collaboration with a French company, Syndivia was able to produce conjugates that have been the subject of a clinical trial on the treatment of blood and bone marrow cancers since 2020. In parallel, the start-up is using other results obtained by Wagner’s team, and in particular a process that enables precise control of the number of drug molecules “attached” to their antibody. They were thus the first to have obtained a “one-to-one” conjugate. “Under this configuration, the coupling is very similar to the antibody alone and will therefore be less rapidly excreted by the body,” explains Koniev. “We have used this process to obtain conjugates that proved highly effective against prostate tumours implanted in animal models,” he adds.
Improved cytotoxic efficacy to limit resistance to treatment
Wagner is also working on the possibility of simultaneously targeting both a tumour receptor and another in the immune system. “We are also trying to graft two active agents onto a single antibody; for example, an active molecule and RNA capable of inhibiting a tumour protein, the aim being to increase the toxicity of the drug.” Indeed, the issue of the efficacy of the cytotoxic agent is crucial because, paradoxically, although in theory the use of a vector enables recourse to drugs that are more toxic than those employed in conventional chemotherapy, the amount actually reaching the tumour is so small that the ais used for targeted therapies need to be extremely potent to exert a tangible effect. Most of the conjugates employed at present to incorporate drugs are based on the same principle – they are tubulin inhibitors. However this poses “problems regarding resistance of the tumours being treated, hence the need to develop new and potent anti-tumour agents whose mode of action is based on other mechanisms,” points out Alami.
For the past fifteen years or so, the scientist has been working on a natural molecule, combretastatin, extracted from the leaves of the Combretum caffrum tree, which is known to have potent anti-tumour and anti-vascular effects. Unfortunately, it is unstable and rapidly transformed into another molecule that is 100 times less toxic. Thousands of analogues were proposed, but no conclusive results obtained. However, in 2007, Alami made a change (patented the same year) to the structure of the molecule that no-one else had considered. And he was right: not only did the ai become perfectly stable but its cytotoxic potency remained intact. This makes the discovery promising, the researcher believes: “This excellent chemical stability, especially in a very acid environment (such as in the stomach) suggests oral administration may be possible, which would be much less complicated for patients than the intravenous route.”
Over the years, the scientists have been refining the properties of their molecule, and have even produced a version with cytotoxic effects that are 100 times more potent than those of the original. “It has proved effective at concentrations lower than 10-9 mole per litre, which is compatible with vectorisation using an antibody.” At the same time, derivatives of the toxic agent that are able to attack both the tumour and its micro-environment have been developed, as have versions capable of stimulating a patient’s immune system. And in 2021, a maturation project with the Paris-Saclay technology transfer company (SATT) led to creation of a start-up, Elikya Therapeutics. “Within this framework, and working in collaboration with Gustave Roussy, our conjugates have achieved some very good results in terms of tumour growth reduction during preclinical trials,” explains Alami. “In the longer term, we hope to be able to supply our molecules under licence to partners specialised in conjugate formulation.”
Second vectorisation strategy: synthetic prodrugs
Alongside antibody-drug conjugates, another type of targeted therapy is also emerging, that of synthetic prodrugs. The idea is to vectorise the cytotoxic agent using synthetic molecules to which it is attached by a covalent bond, rather than resorting to large biological molecules. “The use of ADCs represents an extremely promising therapeutic strategy because they are highly specific regarding their target and are generally well tolerated, although they are relatively costly and complex to produce at this stage. In parallel with research aimed at optimising their pharmacological properties (increasing residence time, improving tumour penetration, etc.), it therefore seems important to explore complementary strategies,” explains Julien Nicolas, CNRS research professor at Institut Galien Paris-Saclay.3

Within the Institute, Patrick Couvreur, recipient of the CNRS Innovation Medal in in 2012, is a pioneer in prodrugs. In the early 2000s, he started working on squalene, a natural lipid present notably in shark liver oil. When squalene is coupled with drug molecules, the resulting prodrug spontaneously forms nanoparticles in an aqueous medium that can incorporate up to 50% of drug mass. In 2017, his team notably demonstrated that once in the bloodstream, these assemblages interact with the lipoproteins that are the natural transporters of cholesterol, for which squalene is a precursor. As a result, they are preferentially vectorised towards tumour cells, which gorge themselves on lipoproteins to ensure their growth.
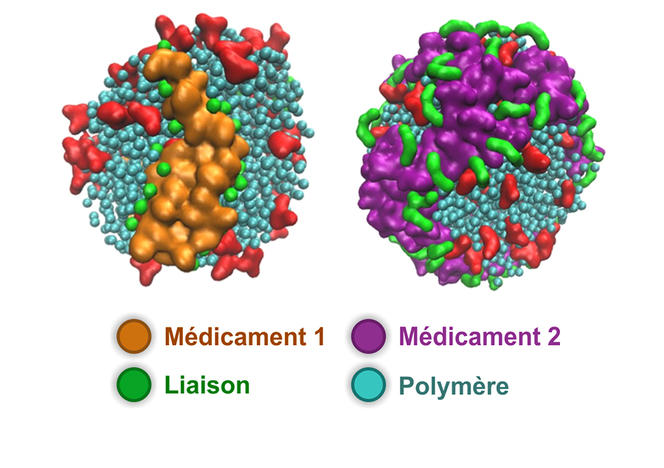
Nicolas, now the leader of this research team, is proposing prodrug nanoparticles formed using biocompatible synthetic polymers. In recent years, his team has been developing a simple and effective synthetic process which consists in growing the polymer in a controlled manner and directly using the active substance. Once the nanoparticles have reached their target, a chemical reaction enabled by the tumour environment cleaves the bond between the carrier polymer and the drug, which recovers its cytotoxic potency. The scientists have thus developed different prodrugs that have demonstrated significant anticancer efficacy in the mouse. Some versions of these assemblages can even transport several active agents simultaneously. “What is interesting about our method is that it is simple and versatile,” Nicolas points out.
Hydrophilic prodrugs to enable activity close to tumours
The chemist has recently developed a hydrophilic version of these prodrugs; rather than forming nanoparticles, the prodrug becomes completely solubilised in blood plasma. “Our idea is firstly, that this may enable a subcutaneous injection that is simple to perform and more comfortable for the patient, and secondly that by acting on the type of chemical bond between the drug and the polymer we can achieve a reservoir effect because of the slow release of the active substance in the body. Indeed, this prodrug can remain in the circulating blood for several days before it is excreted,” he explains. To promote these new concepts even further, in 2019 Nicolas and other colleagues in the laboratory created the start-up Imescia. “The first round of fundraising enabled us to perform a series of preclinical trials in animal models, and we hope to start clinical trials within the next year or two,” he explains.

Sébastien Papot, at the Poitiers-based IC2MP,4 shares the same goal. With the start-up Seekyo (created in 2018), he is currently raising funds to conduct clinical trials of candidate drugs to treat pancreatic and triple-negative breast cancers, for which no convincing treatments exist at present. Their solution is a small, synthetic organic molecule on which it is possible to graft up to three drug molecules and which binds to the albumin in blood plasma so that it can travel into the tumour micro-environment. “Cancer cells are highly heterogeneous so it is often difficult to target them specifically, hence our choice of a so-called non-internalising approach. To date, this has enabled us to treat human lung, colon, pancreatic and triple-negative breast tumours implanted in mice and to achieve unprecedented efficacy,” explains this university professor. While the long term consequences for patients are not yet known, one thing is certain: the CNRS chemists are doing their utmost to contribute to making targeted therapies yet another weapon to fight a disease that remains the leading cause of death in France.
Author
Born in 1974, Mathieu Grousson is a scientific journalist based in France. He graduated the journalism school ESJ Lille and holds a PhD in physics.
Comments
Log in, join the CNRS News community