You are here
Do Black Holes Distort Time?
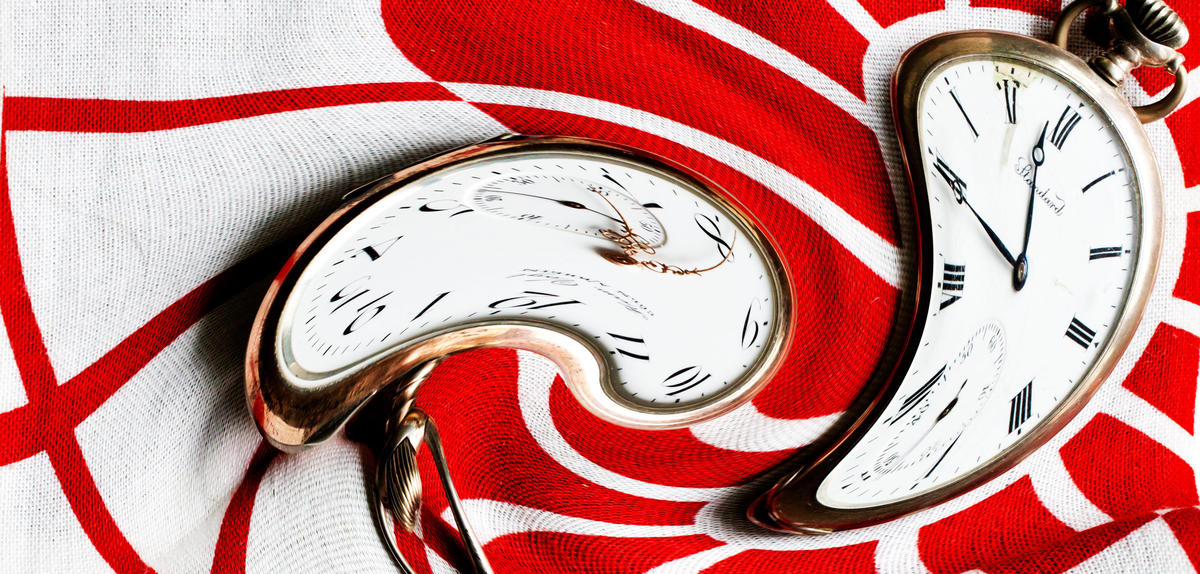
Ten years after his theory of special relativity debunked the myth of the unchanging nature of time, Einstein repeated the feat with his theory of general relativity. In 1905, he showed that a clock on board a moving vehicle 'lost time' compared to a stationary one. And in 1915, he predicted that, in the same way as speed, the gravitational field generated by a massive body would slow clocks down, all the more so as the clock approached the body in question.
More than forty years before the launch of the first satellites, this prediction, known as the "Einstein effect," was as daring as it was difficult to prove, especially as the effect seemed relatively negligible in the gravitational fields generated by the celestial bodies (stars, planets, etc.) observed by the astronomers of the time. And although black holes had been predicted by the equations of general relativity, it was only in the 1970s that their existence was actually proved.
Increasingly accurate corroboration
It wasn't until 1959 that two researchers at Harvard University, Robert V. Pound and Glen A. Rebka, provided the first empirical corroboration of the phenomenon of time dilation through a ground-based experiment. Further confirmation came in 1976, when the Gravity Probe A spacecraft, carrying an ultra-accurate atomic clock, was launched into space to an altitude of 10,000 kilometers. The data showed that, for the instrument, time had speeded up—by approximately 40 microseconds per day at its most distant point—compared with a stationary clock back on Earth. This effect also had to be taken into account when using geo-positioning systems such as GPS, which are based on the comparison of time-series data provided by atomic clocks on board a constellation of satellites. In fact, GPS not only corroborates the theory of general relativity but is also, for now, one of its only applications for the general public.

However, since the slightest departure from Einstein's predictions could open the way to a new physics, physicists are keen to improve the accuracy of measurements as far as possible. It is with this goal in mind that the PHARAO atomic clock will be installed on board the International Space Station in February 2017, at an altitude of 400 kilometers. A core component of the ACES (Atomic Clock Ensemble in Space) experiment, it will be able to measure gravitational redshift with fifty times less uncertainty than that of Gravity Probe A.
"This will be the first time that such an accurate clock has been put into orbit," enthuses Peter Wolf, in charge of analyzing ACES data at the SYRTE laboratory,1 who took part in the design of PHARAO.
What makes this clock so accurate? Like all atomic clocks, PHARAO measures time according to the excitation of atoms, whose oscillations are the equivalent of the ticking of the second hand of a watch. However, in this clock, the atoms "are cooled down by a laser to a temperature close to a microkelvin, which is very near absolute zero," Wolf explains. "This slows their motion, enabling us to observe them better and providing us with a more accurate measurement of time." In addition, a system makes it possible to compare the PHARAO clock with its twins back on the ground via radio. As a result, this "cold-atom fountain" clock will be able to quantify time dilation with a margin of error of only one second in 300 million years.
Black holes, the ultimate test of general relativity
"Einstein's theory works extremely well in a weak gravitational field, for instance when it is studied in the vicinity of the Earth," explains astrophysicist Guy Perrin, "but it hasn't yet been tested in an extremely strong field, such as that found near a black hole." And yet at the heart of our Galaxy, a supermassive black hole called Sagittarius A* is four million times more massive than the Sun.
This will give Perrin and his team at the Laboratory for Space Studies and Instrumentation in Astrophysics (LESIA)2 the chance to confirm the presence of a black hole and to measure its effects in a strong gravitational field, in other words, in the near-immediate vicinity of the object. Located 26,000 light years away, its apparent size in the sky can be compared with that of two one-euro coins on the Moon when seen from Earth. So, as Perrin says, "a very large instrument is the only solution to observe it."
And this is where the GRAVITY interferometer comes in. By combining the light collected by the four telescopes of the Very Large Telescope (VLT) array in the Atacama desert in Chile, the instrument will, as of next November, be the equivalent of a super-telescope 140 meters in diameter. Designed and developed with the participation of LESIA, the instrument will provide a more accurate view of the stars orbiting around Sagittarius A*.

GRAVITY will not only observe the motion of these stars, but also that of potential hot spots. These chunks of matter, heated by the tidal forces exerted by the black hole, emit bright flares near its event horizon. "Even though the resolution is not good enough to allow GRAVITY to map these hot spots, measuring their trajectories will enable us to analyze the space-time metrics in the region." explains Perrin. In other words, the researchers will be able to observe how the black hole distorts both space and time around it, since in Einstein's theory of relativity, space and time form a whole, called the space-time continuum.
Towards a new theory of gravitation?
In order to study the slowing of time caused by Sagittarius A*, the researchers are also investigating another way in which it manifests itself: gravitational redshift. Light waves are stretched out—that is, their frequency decreases—when they travel through the gravitational field of a massive body. "This shows up as a redshift caused by an apparent time dilation, as has been observed in the radiation emitted by iron nuclei in a disc of matter attracted by a black hole," says Éric Gourgoulhon at the Laboratory Universe and Theories (LUTH)3
"Although the theory of general relativity works very well when describing the event horizon of a black hole, it meets its limits when it comes to describing the central singularity, located beyond this horizon," points out Gourgoulhon, a specialist in the behavior of matter in a strong gravitational field and laureate of the 2012 CNRS Silver Medal. "We will therefore need to incorporate general relativity into a more sophisticated theory such as string theory or loop quantum gravity, which we'll be testing in the years to come."
- 1. Systèmes de Référence Temps-Espace (CNRS / Observatoire de Paris / UPMC).
- 2. Laboratoire d’études spatiales et d’instrumentation en astrophysique(CNRS / Observatoire de Paris / UPMC / Université Paris Diderot).
- 3. Laboratoire univers et théories (CNRS / Observatoire de Paris / Université Paris Diderot).
Explore more
Author
Simon Castéran is a journalist with a passion for science. For several years, he has devoted himself to the popularization of science, especially in the field of astronomy, space exploration, and exobiology.