You are here
On the Hot Trail of Cold Plasmas
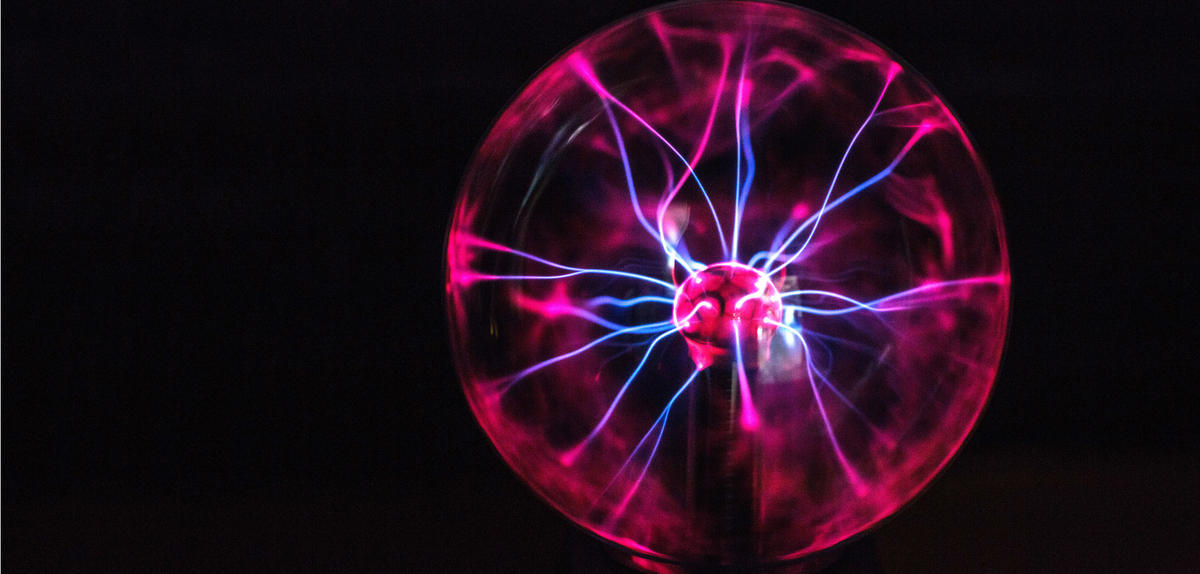
Plasmas are a state of matter of their own, somewhere at the frontiers between science fiction, advanced technology and everyday life. They are the source of starlight, lightning and the aurora borealis, but their power is now being tamed to provide a host of applications that go well beyond flat screen televisions. However, for daily uses, new kinds of plasma that are better suited to human energy and temperature scales have had to be developed: enter the world of cold plasmas.
From lightning to low-energy lighting
Just as ice can be made of water, carbon dioxide, or even nitrogen if you happen to be on Pluto, the word plasma does not refer to a particular substance but rather to the fourth state of matter, along with solids, liquids, and gases. A gas becomes a plasma when enough energy is available to tear the electrons away from its atoms and molecules, thus ionizing it and forming a soup of ions and electrons that can conduct electricity. Stars, for instance, mainly consist of plasma. The latter, which accounts for 98% of the visible matter of the Universe, can reach temperatures of several million degrees. So what does a star have in common with one of those harmless, entertaining plasma globes?
Cold plasmas are obtained by applying an electric discharge to an insulating gas. But, unlike stellar plasmas, only a small proportion of the gas becomes ionized. Some of the electrons are heated to temperatures of several tens of thousands of degrees, but since these electrons only account for a tiny part of the gas, the plasma mostly remains 'cold' on human scales compared to that in stars, which reaches millions of degrees.
The hot, excited electrons in return excite the atoms, which then emit photons that light up the plasma. Fluorescent tubes, low-energy light bulbs and UV lamps are all major applications of cold plasmas. However, plasmas can also lead to the formation of chemical species that some hope to use for health and medical applications in dermatology and oncology for instance.
A novel role in medical treatment
"Research is divided into three main areas: studying the most promising plasma sources, investigating the reactive species, and test the efficiency of the plasma treatment in vivo and in vitro" says Antoine Rousseau, senior researcher at the LPP.1 "In fact, the chemistry produced by the plasma varies from one device to another, but these are phenomena that last around a tenth of a microsecond to about ten microseconds, so it is difficult to quantify and describe the species present. However, we know that some ions and reactive species of oxygen that form in the plasma can react with living tissue."
"Cold plasmas can disinfect wounds and help them to heal," Rousseau adds, "especially in the case of ulcers and chronic bedsores. The mechanisms at work aren't well understood, but biologists believe that certain nitrogen oxides produced by plasmas stimulate cellular responses, and hence tissue reconstruction."
The appliances used in plasma medicine are compact devices containing a high-voltage electrode. The plasma is produced directly when in contact or close to living tissue. The energetic electrons of the plasma cause reactive oxidizing species and ions to form.

Oxidation by plasma can also be used in the field of air treatment, especially to remove pollutants present at very low concentrations, such as formaldehyde and toluene. These can accumulate inside buildings, and it is therefore necessary to remove them by trapping them in a filter. The problem is that molecules found at concentrations below one part per million tend to escape from the filters in the event variations in humidity. The traps are therefore backed up by a catalytic absorbent and a cold plasma: the oxidation of the pollutants by the plasma continuously regenerates the filter.
Improved space propulsion
Plasmas are also present in the electric thrusters used to drive spacecraft and satellites. "We're interested in electric thrusters since they expel matter—in this case ions—at very high speeds, higher than those of conventional chemical thrusters," explains Stéphane Mazouffre, senior researcher at ICARE,2 where he heads the Electric Propulsion team. " We know that faster expulsion allows a lower fuel consumption for a specific maneuver We most often rely on xenon for our cold plasmas, which enables us to halve the weight of a communications satellite, or alternatively, for a spacecraft, to keep the same mass and increase its autonomy."
These engines that operate at low pressure nonetheless produce a relatively weak thrust: for example, the thruster cannot lift off from Earth on its own. Things are quite different when spacecraft are in the zero gravity of space. For instance, satellites are first put in low orbit by a conventional launcher, using chemical propulsion. To place them in their operational orbit and keep them there, cold plasma thrusters then take over.
"The speed of a spacecraft depends not only on the thrust,but also on how long it is exerted," Mazouffre explains. Chemical propulsion systems provide a strong thrust over a very short time interval, whereas cold plasma thrusters produce a weak thrust that lasts a long time. So over greater distances, plasma thrusters can make up for their initial slowness and reach increasingly high speeds: the further, the better."
Extended lifetime wall-less thrusters
Another limitation imposed by space travel, namely the absence of maintenance, is receiving increasing attention. This is because the slightest failure in a spacecraft bound for, say, Jupiter can wipe out fifteen years of work. Researchers are therefore trying to reduce mechanical wear in thrusters, which means, in particular, reducing sources of erosion. One way of doing this is to actually eliminate the thruster's walls.
Prototypes such as a wall-less Hall thruster have recently been developed at ICARE. The plasma chamber is no longer enclosed within solid walls, which would inevitably suffer wear and tear over long space journeys, but is located outside in space, confined solely by a magnetic field. The team can control and adjust the shape of this field, so as to direct the expelled ions and monitor the direction and thrust of the spacecraft or satellite.
Nonetheless, the physical principles underpinning interactions between plasmas, solid surfaces and magnetic fields remain little known. As a result, there is a lack of sufficiently reliable predictive models and simulations, which makes it necessary to carry out testing in a vacuum chamber over very long time periods. Some thrusters are run for 10,000 hours, i.e. around a year, while future tests look set to last for an astonishing 50,000 hours. However, these difficulties should be largely offset by the predicted improvements in speed and autonomy.
Cold plasmas are one of those fields where every new application opens up new avenues. Taking into account the many theoretical aspects that are yet to be elucidated by researchers, it is clear that these advances are still in their infancy, holding out the promise of considerable progress.
Explore more
Author
A graduate from the School of Journalism in Lille, Martin Koppe has worked for a number of publications including Dossiers d’archéologie, Science et Vie Junior and La Recherche, as well the website Maxisciences.com. He also holds degrees in art history, archaeometry, and epistemology.