You are here
Physics to conquer the infinitesimally brief
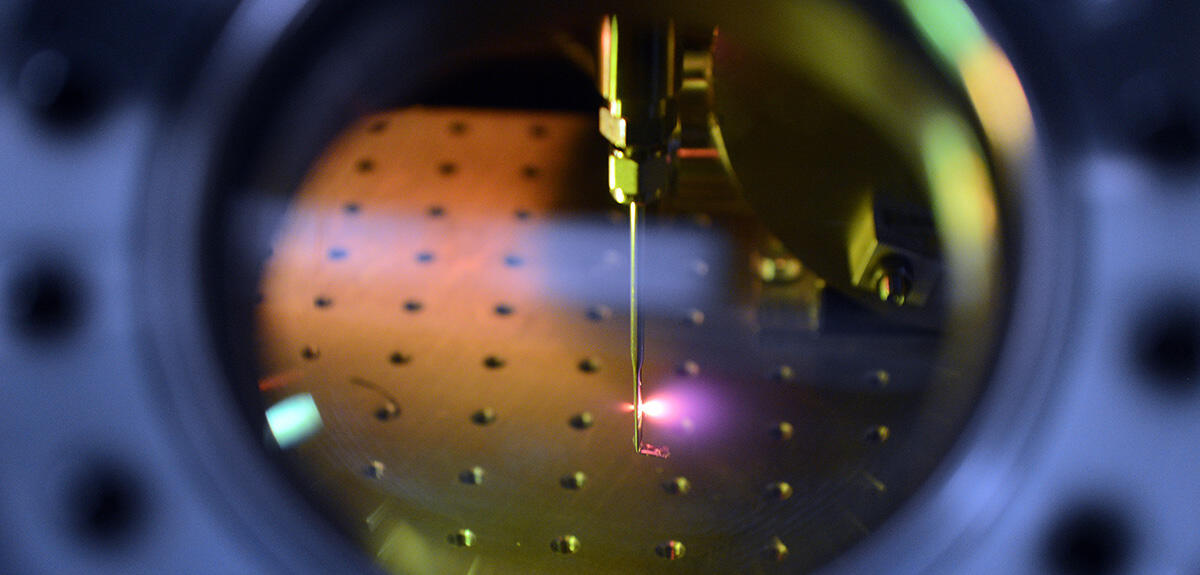
On 3 October, 2023, the physicist Anne L'Huillier was giving one of her regular physics lectures at Lund University in Sweden. During the break she turned on her mobile phone to check her emails – and discovered she had just been awarded the Nobel Prize in Physics, together with the French researcher Pierre Agostini and the Austrian-Hungarian physicist Ferenc Krausz, thus becoming only the fifth woman to receive the distinction, and the second in France, after Marie Curie. But before letting her emotions get the better of her, L'Huillier had some unfinished business to attend to. She resumed her lecture, apologising to her students for having to bring it to an end a little earlier than usual.

The Nobel was awarded by the Royal Swedish Academy of Sciences for experimental methods that generate pulses of light on the order of attoseconds, or one billionth of a billionth of a second (10-18 s). As researchers are keen to point out, there are as many attoseconds in a second as the number of seconds since the Big Bang. These pulses opened up a new field of research: for the first time, scientists had a tool with which to explore ultra-fast phenomena, and in particular electron dynamics.
"When you look at matter, it is the electron cloud that dictates the positions of atoms," explains Valérie Blanchet, a physicist at the CELIA1. "The way in which matter is structured is determined by electrons." Hence the crucial importance of focusing on these tiny negatively charged particles. Attosecond physics brings the “time” dimension to the sciences of the infinitesimally small: it makes it possible to observe not only the initial and final states of a system, but also the transition from one to the other. And that's not all: attosecond pulses can also be used to control electron dynamics in matter. Developed for thirty years by a small group of optics researchers and physicists, attoscience is now attracting the attention of scientists working in many other fields, from chemistry to medicine and biology. It has actually become central to the LUMA exploratory Priority Research Programme and Infrastructure, led by the CNRS and the CEA, which aims to understand, manipulate and harness light to control physico-chemical and biological systems, and pave the way for new green technologies.
Mysterious harmonics
It all started in 1988, when the young L'Huillier, who had recently been recruited by the French Alternative Energies and Atomic Energy Commission (CEA), pointed a laser at some argon atoms. The team she belonged to had a worldwide reputation for research into light-matter interactions. However, this time, instead of observing the effect of light on atoms, she decided to take a look at the photons produced by this interaction. "It was sheer experimental curiosity. No theorist had suggested she should do this," explains Philippe Balcou, a research professor at the CELIA, who, a year after this experiment, was to become L'Huillier's first doctoral student. The future Nobel laureate observed something quite unexpected: the laser triggered the emission of beams of ultraviolet photons whose frequencies were multiples of that of the laser, called harmonics. However, their presence was inexplicable at the time, especially since the intensity of the harmonics, rather than declining rapidly, reached a plateau that extended into the far ultraviolet and even beyond. L'Huillier therefore decided to dedicate her research to this phenomenon.
"At first, these harmonics had everyone flummoxed," admits Richard Taïeb, a researcher at the LCPMR2, and one of the first theorists to work with L'Huillier. In fact, it would take the researchers five years to explain their origin. When electrons are excited by a laser and torn away from an atom (which thus becomes ionised), they escape from its nucleus. However, when the phase of the laser light is reversed, the electron is abruptly pulled back towards the nucleus of the ionised atom, where it then recombines with it. To get rid of the excess energy it accumulated when excited by the laser, it emits an ultraviolet photon. These harmonics rapidly grabbed the interest of experimenters and theorists. "One of the reasons for studying them was to have a coherent light source in the far ultraviolet," Taïeb recalls. In fact, rather than travelling in every direction, these beams are emitted straight back towards the laser. "It was as if we had a kind of pocket synchrotron," says an amused Balcou.
It was in 2001 that Agostini, inspired by the work of the theorists Taïeb, Valérie Véniard and Alfred Maquet, became the first to generate attosecond pulses (lasting around 250 attoseconds), thus heralding the birth of attophysics. The next two decades saw the emergence of more powerful lasers. "When we first started to characterise harmonics in the early 1990s, we had a laser that fired one shot per minute," Balcou recalls. "Today, this exceeds 10,000 times per second." The improvement in lasers went hand-in-hand with better control of attosecond pulse generation. The time had come to put this remarkable tool to good use.
Ejecting electrons
One of the most remarkable achievements of attosecond science was the observation of the photoelectric effect. First described by Einstein in 1905, this refers to the ejection of an electron when an atom absorbs a photon of a particular energy. In 2010, Krausz showed that this phenomenon is not instantaneous, and that the electron takes a handful of attoseconds to escape the attraction of the atomic nucleus. Duration also depends on the characteristics of the excited electron. What delays the ejection? What differences are there between the various electrons in an atom? How do they affect one another? The researchers finally had the experimental and theoretical tools they needed to tackle these questions. “Using attosecond pulses, we were able to resort to a time-resolved approach to start exploring the interactions between electrons, also called electron correlation," Blanchet explains.

Interactions between electrons determine those between atoms, especially during chemical reactions, which always begin with a rearrangement of the electrons: the nuclei only start to move much later. "What I like about attosecond physics is that we can see processes in their very early stages," says Lou Barreau, a researcher at the ISMO3. In her experiments, she uses attosecond pulses to ionise different molecules. "I'm trying to understand how their environment affects the ejection of electrons. For example, does the presence of a methyl group or an aromatic ring influence the time of ionisation?"
By intervening before the chemistry kicks in, the researchers hope to use attosecond pulses to control the course of chemical reactions. For instance, take a molecule that needs to be split at a specific point in order to obtain a particular product. "The idea is to excite the electrons in a molecule so as to create an electron wave. The wave would propagate through the molecule and weaken certain atomic bonds," Barreau explains. In this way, attosecond pulses would be used to “etch” lines of weakness in the molecule before the chemical reaction takes place, in order to obtain the desired products. However, a lot of work will be needed before these new possibilities can be put into practice. "We already have proof of principle using amino acids, but for the moment they are in the form of ions. We haven't yet succeeded in doing this with neutral molecules," Barreau admits.
Attosecond researchers have also shown great interest in what are called chiral molecules. These come in two versions, called enantiomers, which, like our two hands, are absolutely identical except that they are mirror images of each other. Since the nineteenth century, it has been known that enantiomers are optically active, meaning that they can rotate the plane of polarised light in two opposite directions. The HXUV team at the CELIA have ionised chiral molecules and shown that the time taken for electrons to escape from them and the direction in which they are ejected depend on that of the polarised light as well as on the enantiomer.
The inexorable expansion of attosecond science
Attosecond physics is also proving to be of interest for other disciplines. "Advances in attophysics now make it possible to study more complex objects that connect us to other fields," explains Franck Lépine, a researcher at the Institute for Light and Matter (ILM)4. One such field is astrophysics. "Molecules in the interstellar medium react to the ionising radiation present in space," the researcher points out. In order to better understand the relationship between interstellar chemistry and ionising radiation, his team used attosecond pulses in the far ultraviolet to study the stability of carbonaceous molecules, the precursors for the emergence of life in the Universe.
He also relies on attosecond methods to study DNA and proteins. "We are focusing on the damage that high-energy radiation causes in living matter. Understanding the initial stages of such processes could help us to come up with new ways of protecting ourselves from this radiation," says Lépine, who is developing a new method for early medical diagnosis in collaboration with Krausz. "Using broad-spectrum attosecond pulses, we can detect minute traces of certain molecules that signal the presence of cancer in biological fluids, and thus predict the onset of the disease," the physicist explains.
The researchers believe that it will still be several years before the attosecond plays a role in everyday life. "I compare the attosecond domain to that of lasers. The concept of the laser was first put forward in the early twentieth century. It then took fifty years to build the first one, and another thirty years for it to revolutionise our everyday lives with barcodes, CDs, and laser surgery," points out Fabrice Catoire, a theorist in coherence at attosecond scales at the CELIA. However, even though it will be some time before the attosecond affects our daily lives, it is highly likely that it will soon become part of the scientists' toolbox. "You only have to look at the way in which the femtosecond5 and picosecond 6 domains have progressed since the 1980s and 1990s," Lépine points out. "From very basic things, we moved on to applications in industry, surgery, and ultra-fast spectrometry. Here we’re talking about femtochemistry and femtomagnetism. In the attosecond domain, we're at the proof-of-concept stage, but little by little we’ll be branching out into other areas." In conclusion, we'll just have to be patient and remember that scientific breakthroughs don’t happen in an attosecond! ♦
-----------------------------------------------------------
Laserix and the French exception
The string of French physics Nobels in recent years (Gérard Mourou, Alain Aspect, Pierre Agostini, Anne L’Huillier) is no coincidence. Their careers have all been marked by their intensive use of lasers, a field in which France has consistently stood out since the 1960s thanks to a unique combination of high-level technicians, engineers and researchers, platforms of excellence and world-beating companies in the sector, such as Thales and Amplitude.
Laserix, the laser platform at Université Paris-Saclay, is part of this success story. Since 2003, it has been offering researchers from a wide range of disciplines femtosecond laser sources of exceptionally high quality, using the artist-in-residence model. It specialises in the generation of pulses at very short wavelengths in the far ultraviolet, and "provides a source with 10 to 100 times more energy than harmonic sources at these wavelengths", says Sophie Kazamias, scientific director of the platform since 2015.
One area of research at Laserix is laser-plasma acceleration. This technique uses accelerator fields 1,000 times more powerful than those of today's machines to boost electrons up to speeds close to that of light. At such velocities, relativistic phenomena start to appear, such as a significant increase in the mass of particles. This is undoubtedly one of the hottest topics in physics today. "Laser-plasma acceleration will one day be honoured with a Nobel Prize. And I bet you anything that one of the laureates will be a French scientist," predicts Kazamias. ♦
- 1. Centre Lasers Intenses et Applications (CNRS / CEA / Université de Bordeaux).
- 2. Laboratoire de Chimie Physique-Matière et Rayonnement (CNRS / Sorbonne Université).
- 3. Institut des Sciences Moléculaires d'Orsay (CNRS / Université Paris-Saclay).
- 4. CNRS / Université Claude Bernard Lyon 1.
- 5. 1 femtosecond = one millionth of a billionth of a second (10-15 s).
- 6. 1 picosecond = one thousandth of a billionth of a second (10-12 s).