You are here
Is CO2 capture the key to carbon neutrality?

(This article was originally published (in French) in issue 16 of the CNRS journal Carnets de Science)
One of the central messages in a European Commission report published at the beginning of February 2024 laying down broad guidelines for industrial carbon management in Europe is that, if we are to limit global warming to 1.5 °C, we will have to rely on the capture and storage of carbon dioxide (CO₂)1. According to the Commission, carbon capture and storage (CCS) will be key to removing around 280 million tonnes of CO₂ per year from the atmosphere by 2040, rising to some 450 million tonnes by 2050. This goes to show just how much Brussels is banking on this approach. However, the trouble is that, as an article published a few days later in the journal Nature2 pointed out, the European Union's dependence on this technology is not without its dangers. And for good reason: the roll-out of CCS is encountering a number of obstacles.
The 2023 report published by the Global Carbon Project3 (an organisation that seeks to quantify global greenhouse gas emissions and their causes), estimates that CO₂ emissions caused by human activity (industry, transport, heating, etc.) amount to some 40 billion tonnes annually. CO2 is the principal greenhouse gas contributing to global warming, and according to the Intergovernmental Panel on Climate Change (IPCC), a temperature rise in excess of 2 °C would lead to a significant increase in sea levels and to more frequent extreme weather events such as floods, storms and droughts.
Capturing residual emissions
To achieve carbon neutrality in 2050, the IPCC is focusing on two main solutions: firstly, reducing emissions by cutting energy consumption, using energy more efficiently, and gradually replacing most fossil resources (oil, gas, etc.) with zero-carbon energy (solar, wind, nuclear and tidal); and secondly, removing CO₂ from industrial flue gases, or even directly from the air.
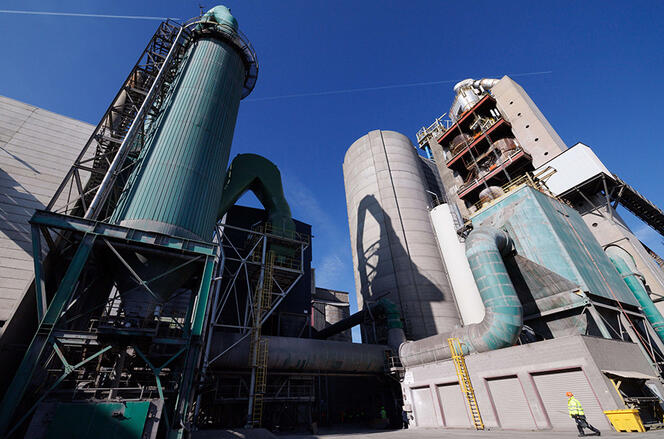
However, the latter option is struggling to get off the ground – the largest facility in operation to date is capable of capturing a mere 4,000 tonnes of CO₂ per year, a drop in the ocean compared to annual global emissions. However, this is not the case for the direct removal of carbon from flue gases, especially those resulting from the chemical reactions needed to produce various materials. For example, while one third of the CO₂ emitted by a cement works comes from the combustion of fossil fuels in its kilns, the remaining two thirds is produced by the thermal decomposition of limestone, the main raw material for cement.
These residual emissions can be captured by many natural carbon sinks, such as oceans, forests or peatlands, which sequester CO₂ in the form of organic matter thanks to the biochemical process of photosynthesis. However, these ecosystems only trap just over half of today's CO₂ releases, which is already a huge amount. But with accelerating climate change, there is a risk that carbon sinks will become less effective, particularly if their surface area shrinks due to fires, drought, and loss of natural and semi-natural land to urban and other artificial development (known as “land take”). Hence the need to capture CO₂ artificially.
"Although natural carbon sinks will be able to reduce residual emissions by 80% by 2050, most experts believe that CCS will be essential for the remaining 20%," says Jacques Pironon, research professor at the GeoRessources4 laboratory in Nancy (northeastern France), who is involved in CCS research.
Burying smokestack gases underground
Specifically, CCS aims to trap the carbon dioxide contained in flue gases directly from the smokestacks, and then store it underground. In practice, "there are three major stages in CCS: capture, transport, and storage of CO2, all of which are based on specific technologies", explains Florence Delprat-Jannaud, president of Club CO₂, which promotes discussion and initiatives among industry and research players concerned by CCS. To date, there are three main approaches to CO2 capture. "The most mature is post-combustion, which aims to extract carbon dioxide from flue gases derived from combustion. It's been used for a century by the petroleum and gas industries," says Florent Guillou, a design process engineer at IFP Énergies Nouvelles. In this approach, CO₂ is absorbed by amine-based solvents, compounds that can bind to the gas.
A second approach is pre-combustion capture, which "consists in removing CO₂ from the fuel before it is burnt. This is done by transforming the combustible into a synthesis gas, composed of carbon monoxide and other gases, and then injecting steam in order to turn part of the energy source into CO₂, with the additional production of hydrogen". Finally, the third approach, CO₂ capture by oxycombustion, is a technique based on using pure oxygen instead of air to burn fuel during industrial processes that require combustion. "This produces a flue gas containing almost nothing but CO₂ and water vapour, which can be easily separated," Guillou explains.
Recycling oil and gas fields
To transport the captured CO₂, "the gas is liquefied in order to reduce its volume, and then taken to the place of storage by pipeline, ship, train or lorry", Delprat-Jannaud adds. This is followed by the storage stage, which “can be carried out in various underground structures located at depths of over 800 metres, such as deep saline aquifers (beds of porous, permeable rock saturated with saltwater unfit for drinking), depleted oil and gas fields, and igneous rocks like basalt and peridotite".
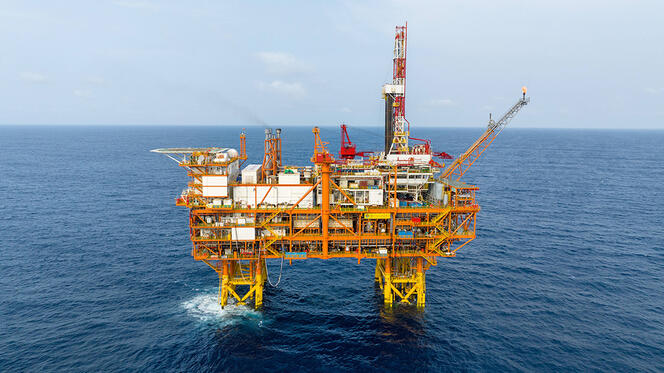
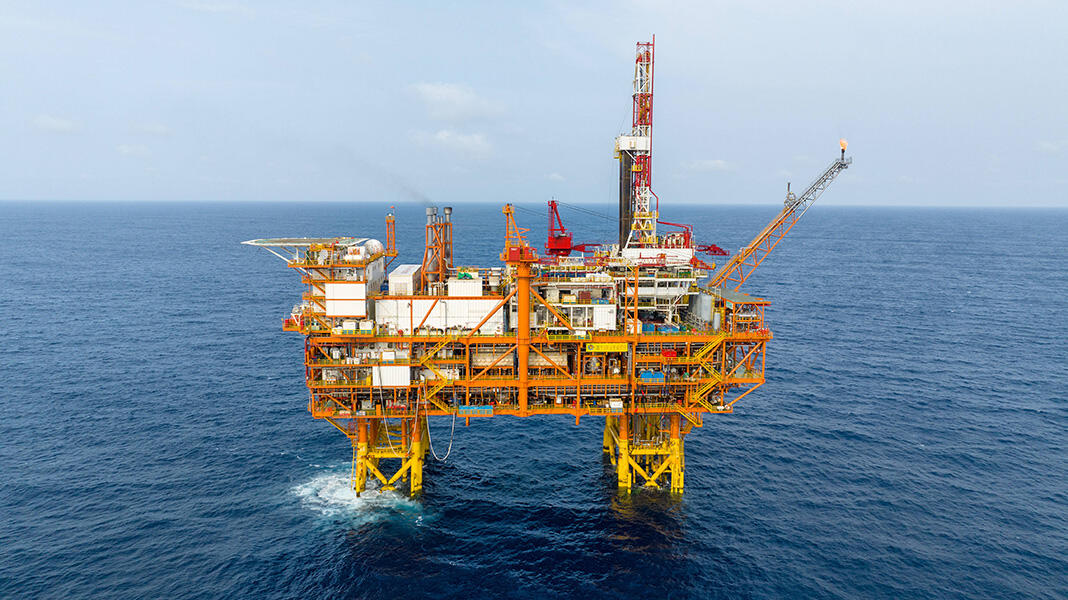
For Pironon, "former oil or gas fields are the easiest option to implement, since CO₂ can be injected through existing wells. In addition, for several decades the oil industry has been using enhanced oil recovery, a process whereby CO₂ is introduced into reservoirs at the end of their life in order to reduce the viscosity of the hydrocarbons and thus recover them more easily". Yet "saline aquifers are far more numerous and provide the greatest storage capacities", Delprat-Jannaud stresses. According to the IPCC, these geological formations could contain 10,000 billion tonnes of CO₂ worldwide, enough to store all our CO₂ emissions for centuries to come.
As for igneous rocks, "they provide long-term storage capacity via mineralisation of CO₂ into carbonates, in other words, very rapid solidification and stabilisation in the rock in just a few years, as compared to several hundred thousand years in deep saline aquifers", points out Pascale Bénézeth, research professor at the Géosciences Environnement Toulouse laboratory5 who is studying this option.
Large-scale deployment of CCS
In fact, CCS is not a new technology. "Since the 1990s, Europe has backed a huge number of pilot research projects," says Pironon, who in the early 2010s helped to evaluate the world's first integrated industrial chain for CO₂ capture, transport and storage, tested on the Old Continent at the pilot plant developed by TotalEnergies at its industrial site in Lacq, in southwest France. "Today, there are a total of 41 whole-chain CCS projects worldwide. Better still, Europe's first commercial transport and storage scheme will soon be up and running: Norway's Northern Lights site aims to store 1.5 million tonnes of CO₂ per year under the North Sea from 2024, rising to 5 million tonnes from 2026," Delprat-Jannaud rejoyces.

However, she sounds a note of caution: "Only around 45 million tonnes of CO₂ are captured each year worldwide, whereas in ten years' time it will be necessary to trap between 50 and 100 times that amount in order to achieve carbon neutrality." Hence the need for very large-scale deployment of CCS. For instance, "in the case of France, which aims to store an annual 15 to 20 million tonnes of CO₂ by 2050, the number of sites required will of course depend on the capacity of the facilities, but taking the storage volume of the Northern Lights project as an example, we would need around ten installations of this type. And we would also have to pool them, along with transport infrastructures". Unfortunately, however, the deployment of CCS is being hampered by a number of barriers.
In work published in 20216, a team led by Xavier Arnauld de Sartre, a research professor at the TREE energy and environmental transitions laboratory7 at Université de Pau (southwestern France), identified several types of obstacle by studying the specific cases of some ten European storage projects, based on press articles and social science research.
Social, technical and political hurdles
One interesting finding is that the deployment of CCS is hampered by its low acceptability by the population. "However, this factor only plays a minor role in delaying its deployment," the researcher points out. "Public opposition is partly due to the potential dangers of the technique, such as possible earthquake hazards if the injection of CO₂ is too quick, or the risk of gas leakage." Yet according to the IPCC, "geological sequestration is reliable, with overall leakage rates below 0.001% per year". According to Arnauld de Sartre's team, another source of CCS's difficulties is its lack of maturity. "On the TRL (Technology Readiness Level) scale, which assesses the maturity of a technology up to its integration into a complete system and its industrialisation, geological storage is ranked between 6-7 out of 9," the researcher adds.
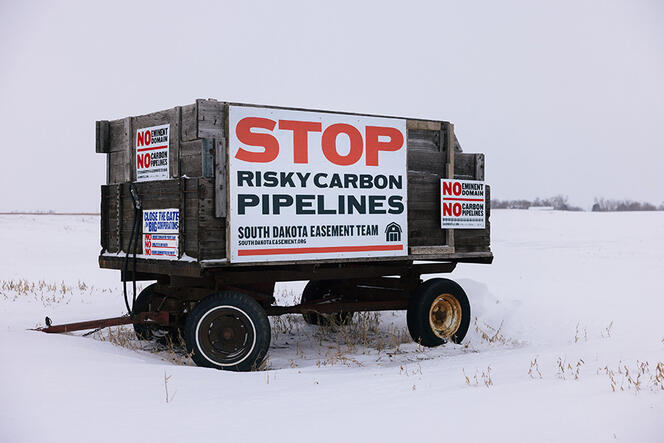
As far as capture is concerned, "there is still a need to improve the efficiency of existing techniques and reduce their cost, especially in terms of energy consumption", Pironon believes. In fact, at the present time, "every tonne of CO₂ captured, transported and stored using CCS costs between €80 and €150, whereas the value of a tonne of CO₂ emitted (paid by an industrial operator if they exceed the authorised limit) is around €90 on the European market", Delprat-Jannaud points out. On the storage side, precise identification of potential reservoirs will be crucial. With specific regard to the igneous rock option, "the challenge is to identify an initial pilot site in France. For instance, Réunion and New Caledonia are potential targets to be evaluated", explains Isabelle Martinez, a researcher at the IPGP institute of Earth physics8.
Finally, the work of Arnauld de Sartre and his colleagues highlights several other major obstacles slowing down the deployment of CCS: "a lack of agreement of the various players involved concerning the technologies and development strategies to be used; the absence of an economic model specifying who is to pay for storage; and finally, the weak political commitment to CCS". Regarding this last point, the team came to the conclusion, in other research currently in the process of being published, that "due to France's deindustrialisation and the significant development of carbon-free energy provided by nuclear power, the State has invested very little in decarbonisation". However, as Delprat-Jannaud notes, "there will be no other choice to combat climate change but to deploy CCS on a grand scale. Fast!" ♦
- 1. "Communication from the Commission to the European Parliament, the Council, the European Economic and Social Committee and the Committee of the Regions – Towards an ambitious Industrial Carbon Management for the EU", European Commission, 2 February 2024.
- 2. doi: https://doi.org/10.1038/d41586-024-00391-3
- 3. https://essd.copernicus.org/articles/15/5301/2023/
- 4. CNRS / Université de Lorraine.
- 5. CNRS / CNES / IRD / Université Toulouse III – Paul Sabatier.
- 6. S. Chailleux et al., Natures Sciences Sociétés, December 2021. https://doi.org/10.1051/nss/2021018 (in French).
- 7. CNRS / Université de Pau et des Pays de l’Adour.
- 8. CNRS / Institut de Physique du Globe de Paris / Université Paris Cité.
Keywords
Share this article
Author
A freelance science journalist for ten years, Kheira Bettayeb specializes in the fields of medicine, biology, neuroscience, zoology, astronomy, physics and technology. She writes primarily for prominent national (France) magazines.
Comments
Log in, join the CNRS News community