You are here
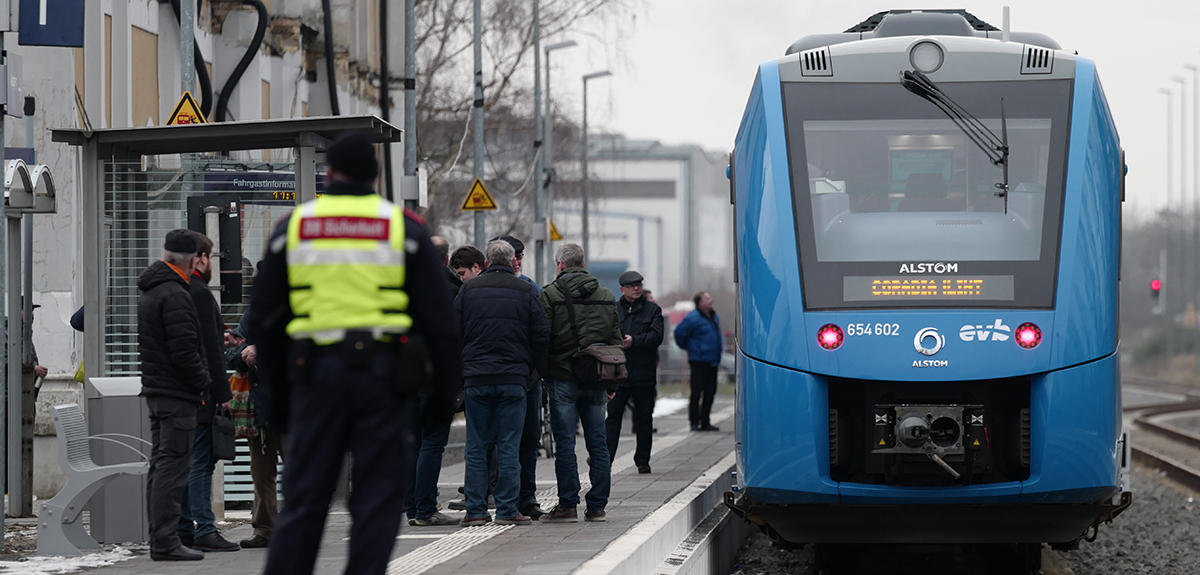
Hydrogen is the most abundant atom in the Universe, and therefore represents a potentially unlimited resource. How can energy be extracted from it?
Daniel Hissel:1 Hydrogen is not a primary source of energy, but rather an energy vector. It must first be transformed into dihydrogen, its molecular form (H2), so that it can be transported, stored, and ultimately used to draw energy. There are two primary methods for doing so. The first consists in burning it in turbines, like a traditional fuel. The advantage is that, on an equal weight basis, it will enable three times more thermal energy to be released than petrol. What's more, this combustion is decarbonised, as it only produces water, and no carbon dioxide (CO2). As a result, dihydrogen is being considered for consumer aviation, as it would allow "clean" planes to fly, although nitrogen oxide and other pollutants are emitted during combustion.
The other method, which we are working on in our laboratory, is to use hydrogen fuel cells to produce electricity and heat. In short, such cells involve a reaction between dihydrogen and oxygen: the dihydrogen molecule is dissociated, and hydrogen atoms combine with oxygen from the air to form water. There is also the emission of heat and an exchange of electrons, which generates an electric current.
Lets talk about H2 itself. It is not naturally present in nature but must be produced. How is that done?
D. H.: The most common method today is the reformation of methane (CH4) using overheated steam. The methane and water combine to form both H2 and CO2. In general, 95% of H2 is produced in this way by resorting to fossil fuels such as gas, petroleum, and – worse still, coal. This is a shame, for while the electricity generated by hydrogen fuel cells is green, it is obtained from "grey" H2, which only shifts the problem. It is therefore crucial to "green" the sector.
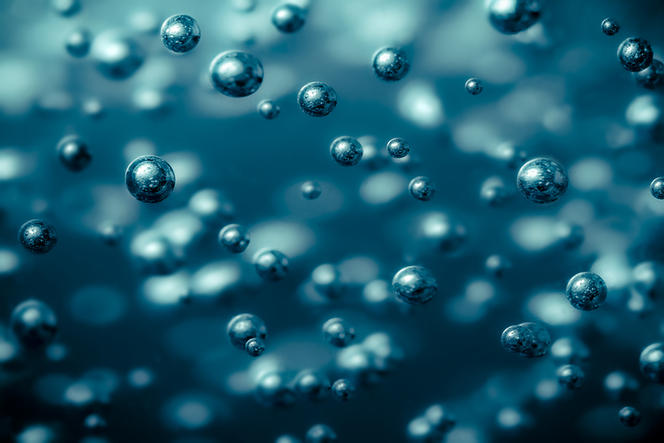
To do so, there is a well-known technique, known as electrolysis, which simply consists in producing the opposite reaction to the one that occurs in the fuel cell. In plain terms, passing an electric current through water makes it possible to obtain dihydrogen and oxygen. Today electrolysis represents a mere 5% of H2 production. This proportion can of course be increased by manufacturing more electrolysers, but this raises the question of the electricity source powering them, which must be renewable – either solar or wind. That said, the electricity in the French network is already highly decarbonised, since it is primarily of nuclear origin. Of course it would be ideal to expand the amount of renewable energy in the French mix, but this challenge exceeds the framework of the hydrogen sector. In any event, we are not yet in a phase of energy degrowth, so we must keep in mind that replacing fossil fuels with hydrogen produced through electrolysis will mean a rise in global electricity production.
Fuel cells were invented in 1839. Shouldn’t there be one in every home today?
D. H.: If this is not so, it is because for a long time, other types of generators, such as electrochemical cells and batteries, were preferred, and efforts to develop hydrogen fuel cells were sporadic. But it is worth looking back at the changes that have occurred over the last twenty years. When I began working on the subject, fuel cells were extremely expensive, with a short lifespan and weak performance. Our laboratory's efforts, as well as those of all players in the field, including industrial actors, have focused on these three aspects. As a result, for the same amount of power, the price today has been divided by thirty, and the volume required by fifty. Lifespan has been multiplied by 40 or 50, depending on the use.
What actual performance have all these division and multiplication factors made possible?
D. H.: The US Department of Energy (DOE) established a performance benchmark for the automobile transportation industry, by imagining the replacement of combustion engines with fuel cells, on the basis of an annual production of 500,000 units. To make this replacement possible, a lifespan on the order of 5,000 to 8,000 hours, or 400,000 kilometres, would be necessary. Its density would have to be under 1.5 kW/l (the volume of a fuel cell producing 1.5 kilowatts must be less than a litre). With regard to energy conversion efficiency, 60% of the chemical energy of H2 molecules must be converted into electrical power.

Finally, the cost must remain below 40 €/kW, as approximately 30-40 kW are needed to equip a car, equivalent to about 1,600 € in the case – used as a reference – of electric hybridisation. So where do we stand today? The density target has been met. Durability now reaches 4,000 hours, and 55% efficiency has been attained. The price comes out to €50-60/kW for an annual projected production of 500,000 units. In short, all of the objectives have either been achieved or are in sight.
How do you explain such progress?
D. H.: Well, first, there was plenty of room for improvement! There were also numerous factors of progress, half of which came from basic research, and half from the optimised management of fuel cells, for example via artificial intelligence. Upstream research enabled progress in the field of materials, in particular nanostructured materials that reduce the volume of fuel cells. The membrane, in which electrons are exchanged, also improved greatly with the development of new polymers. The use of platinum, an expensive but indispensable catalyst, was optimised. That’s about it concerning basic research. Then there is the integration of cells within their applications, which is what we focus on here.

This specifically involves improved management of fuel cells, for instance by enhancing energy performance and durability. We are working on all of the auxiliaries necessary to their functioning: electrical energy converters, cooling systems, real-time diagnostics of battery health, and sensors providing estimates of their remaining lifespan. Beyond the progress made on the cell and its use, there has been growing awareness of the need to reduce greenhouse gas emissions. Industrial actors – car manufacturers in particular – have decided to invest in this promising sector. What's more, the maturity of this technology enables other applications, which further encourages industrial actors considering investment.
The traditional catalyst in fuel cells is platinum, a precious metal worth the price of gold. Isn't this an obstacle to the widespread use of these cells?
D. H.: Today's internal combustion vehicles already include between 2 and 8 grams of platinum, which is not negligible, in the "catalytic" converters of their exhaust pipes. A fuel cell for a car would need between 10 and 20 grams. This is still too high, but we hope we can lower this amount. Besides, platinum is one of the precious metals that can be efficiently recycled on a global scale, thanks to the catalytic converter. So the answer is no, the use of platinum is not an obstacle at present, although its recycling should be improved.
Are there other barriers that must be broken down?
D. H.: I wouldn’t speak of barriers, for it entails a "blockage". And there is no such blockage, for industrial applications already exist. Progress is nevertheless needed, especially in terms of cost. Storage must also improve. While it is not problematic in "stationary" applications where there is room, it is more difficult in restricted spaces such as cars. Achieving comparable independence to that of internal combustion vehicles, in other words 600-700 kms, would require pressurised hydrogen tanks (at 700 bars) that are relatively bulky and not easily configurable. A hydrogen tank is a gas cylinder. It is out of the question to mould it out of plastic, like a gas tank. This is a major constraint when it comes to designing a car. The model sold by Toyota includes two cylinders measuring 40 centimetres in diameter and one metre in length. They are sizeable, but we can make do. The car even has a boot! However, for aeronautics applications for example, in which the on-board quantities are much larger, there is no choice but to liquefy the hydrogen. Doing so requires high pressurisation and cooling to -253 °C. Maintaining this temperature with minimal energy loss is no mean feat.
Hydrogen production methods will surely impact price, and hence the sector's viability.
D. H.: Regarding the price, the DOE also set viability targets: hydrogen at the pump should cost approximately €3 per kilo (allowing a car to travel approximately 100 km). For now, this varies between €2 for carbon-based hydrogen and €6 for its green equivalent from electrolysers. The price of green hydrogen should therefore be halved, which is not unrealistic. For that matter, we have every reason to develop a dedicated sector here in France. Since the start of the Covid-19 crisis, there has been much talk about onshoring. This is an opportunity to produce a perennial French energy that generates no greenhouse gas emissions, and can be widely used to replace petrol, diesel fuel, and fuel oil, among others.
In what fields will fuel cells be found in the future?
D. H.: They're already being used. There are commercial applications for non-subsidised hydrogen fuel cells that are much more competitive than those they have replaced. I am thinking, for example, of the electric forklifts in warehouses, which will eventually be powered solely by "hydrogen" electricity. And functions are not limited to mobility (cars, buses, lorries, trains), but are also "stationary". They include electricity production at isolated sites through generators – without the noise or pollution; or equipping a house or building with a fuel cell that produces both electricity and heat, for it yields water at a temperature of 80 °C, which can be used as it is for heating.

Hydrogen, which is highly inflammable, can deter future users. What about security?
D. H.: Hydrogen stores energy – and all stored energy must be handled with care. This is true of both a petrol tank and a gas cylinder, for instance. Is a hydrogen tank more dangerous? Not necessarily, according to the French National Institute for Industrial Environment and Risks (INERIS), which in recent years has conducted several studies with crash tests, live gunshots on tanks, and various kinds of leaks, among others. Hydrogen is a gas that is lighter than air, and therefore always moves upwards in case of a leak, unlike LPG or diesel fuel, which accumulate under the vehicle, to take one example from transportation. However, it does present an additional constraint in comparison to these fuels, as it is highly compressed in the cylinder, which can cause major explosions. For now the feedback on tanks already in circulation is positive, with no serious accidents reported. So yes, it must be handled with care, as do other forms of stored energy.

What is France's position in this emerging hydrogen economy?
D. H.: We are among the frontrunners, which is good news, along with China, Japan, and South Korea, to name but a few. In Europe, Germany and the Nordic countries, Norway in particular, also rank high. The United States is also present in this field, albeit behind Canada. So we are in good place, but we should be careful, as the strategic choices that we make now will determine our position in the next five to ten years. The sector received a boost in the early 2000s with substantial funding for research, but nothing since. It is crucial to plan out the French effort for hydrogen. The required investments are massive, and industrial actors will not risk it without a minimum amount of visibility concerning the political, environmental, and regulatory stakes involved.
As it happens, last 9 September the French minister of the Economy, Bruno Lemaire, presented the country’s plan for developing decarbonised hydrogen. Seven billion euros will be invested over a decade, including €2 billion in the next two years.
D. H.: This plan sends a clear message to both scientists and industrial actors, which is: "count us in". The €7 billion envelope is significant, but we're not sure how it will be allocated. For the first time, it covers the entire sector, ranging from industry to research (€65 million) at the other end of the chain, in addition to professional training (€35 million). Yet this initial impetus must be confirmed in the coming years, with a dual focus: the pursuit of investments of course, as well as the political commitment to support the sector at the local, national, and European level. This will require an appropriate legislative framework. As an example, if only electric vehicles are to access city centres in the future, local authorities will have to provide hydrogen buses, waste containers for hydrogen, etc. If these buses are bought on a massive scale, they will quickly get economically competitive compared to diesel versions. This is how a market will be created, and how the use of hydrogen will become widespread.
- 1. Professor at the University of Franche-Comté, deputy director of the CNRS Research Network on Hydrogen Energy (FRH2), and manager of the Hybrid Electric Systems, Electric Actuators, Fuel Cell Systems (SHARPAC) team at the Femto-ST (“Franche-Comté Electronics Mechanics, Thermal Science and Optics – Sciences and Technologies”) Institute (CNRS / Université de Franche-Comté / Université de Technologie Belfort-Montbéliard / ENSMM).
Keywords
Share this article
Author
After studying physics, Fabrice Nicot turned to scientific journalism. For 17...
Comments
Log in, join the CNRS News community