You are here
A Bright Future for Solar Energy?
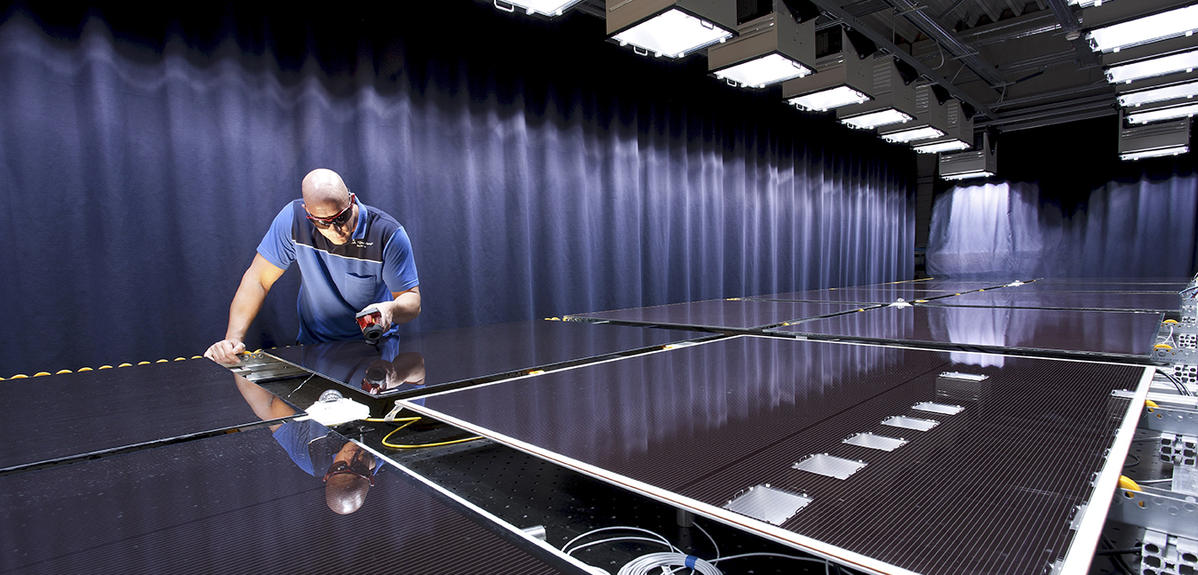
The photovoltaic effect, discovered in the 19th century, is partly based on the properties of semiconductor materials used in solar panels. How are you working to improve these materials?
Daniel Lincot:1 Research on photovoltaic solar energy involves teamwork. The aim is to manufacture more efficient cells, which convert sunlight to energy more effectively, while also reducing costs. To increase the efficiency of photovoltaic cells, you have to improve the semiconductor properties of each material present in the cells individually, such as silicon, but also the combinations of these materials. In some ways, a photovoltaic cell is like a soccer team: it must form a coherent unit, in which the team combines individual elements successfully. The materials must be excellent individually, but they must also be able to transfer electrons back and forth.
Pere Roca i Cabarrocas:2 In the quest to improve efficiency and reduce costs, the slightest detail counts. Research on photovoltaic solar energy is in reality an open-ended dynamic field that incorporates physics, electronics, chemistry, materials science and optics. To improve solar cells, we really need a multidisciplinary approach.
D. L.: And research is quickly moving forward. When the first silicon cells were developed in the 1950s, their efficiency rose within a few years from 0% to over 10% and up to 25.6% today. The current view is that this figure could one day approach the theoretical value of 85% ! This means that there’s still considerable room to grow in the photovoltaic field.
Each day, the Sun provides the Earth with an average 3kWh of energy per square meter. This energy source appears limitless. So what bridles still limit its large-scale development?
P. R. C.: Contrary to general belief, solar energy is already a viable and competitive form of energy. In France, the national electricity provider EDF encourages its consumers to become standalone solar electricity producers. And in many other countries, the Sun is a competitive energy source with respect to grid electricity.
D. L.: Practically all bridles have been removed worldwide. The solar-powered test airplane Solar Impulse has single-handedly shown how some key energy storage problems can be overcome and how daytime and nighttime supply can be alternated. All of these bridles have been removed simply through demonstration. The only remaining obstacle is that we still need to explore much further along this path… In France, a number of economic, political and cultural constraints continue to hinder our progress.
P. R. C.: Solar Impulse showed that solutions exist, and that with intelligent energy management we will find them. There exist even more convincing examples—though less covered by the media—proving the potential of photovoltaic energy. We can imagine solar energy being harnessed by the southern countries to generate wealth through commercialization of part of their production. This dream could actually become a reality.
Silicon, which has been used since the 1960s to power satellites in space, is today the key material in solar panels. Why is it not yet perfect?
D. L.: The silicon sector represents about 90% of the solar energy market. It is based on a simple principle consisting in slicing silicon ingots into wafers to form photovoltaic cells able to transform solar light into electricity. The problem is that silicon needs to be rather thick to completely absorb sunlight.
P. R. C.: The highest efficiency of silicon measured in a laboratory is 26.33%, but we need to do better than this. We know that it is possible to achieve efficiency in excess of 60% using advanced concepts such as multi-junctionsFermerstacking of several semiconductors. In any event, there is nothing in the principles of physics to prevent us. The more effectively we trap photons, the better the efficiency
Does this mean that we need thinner materials to improve the efficiency of solar panels?
D. L.: It’s not really a question of efficiency, but rather one of procedures and costs. This is the entire issue at the heart of the so-called Thin Film PV sector, which accounts for about 10% of the market. It comprises three types of semiconductor materials: cadmium telluride, CIGS3 and thin layers of silicon. The process involves covering a support with a thin layer of one of these materials, making the photovoltaic cells themselves 100 times thinner, and thus potentially less expensive.
P. R. C.: The thin-layer silicon sector is extremely encouraging. The different thin film PV modules are already achieving efficiencies between 12 and 15%.
D. L.: Moreover, thin-film technology is present in everyday life. For instance, the inside of your bag of chips is coated with a thin layer of aluminum, which protects the contents by keeping out oxygen.
In addition to the issue of efficiency, are you not also seeking to reduce costs to make solar energy available to all?
P. R. C.: The aim of solar research is to make better equipment more cheaply.
D. L.: Our aim is to manufacture renewable energy at low cost. Not everyone can afford the technology used in Solar Impulse! But today’s prices will not be the same tomorrow. For example, flat screens were extremely expensive to begin with, but today everybody has several of them. A few years back, photovoltaic modules were 5 to 10 times more expensive than they are now.
Do any of the emerging sectors strike you as more promising than others?
D. L.: Aside from silicon and thin layers, there are other sectors, though less developed, and rather in the emerging stage, such as PV with concentrated light. The multi-junction cells used are coupled with mirrors or lenses that concentrate the energy reaching the cells, which track the Sun’s path. The record efficiency of concentration cells is 46%, but their manufacturing cost is still very high.
P. R. C.: Concentration photovoltaic energy modules produce more Watts using smaller cell surfaces, but they can only use "direct" solar radiation and can only operate without cloud cover. For solar energy, we are always seeking to establish which material will come out on top, but this depends to a great extent on the conditions of use. The efficiency of each sector depends on whether the operating conditions are cold, hot or wet. Ultimately, I think the ideal approach is to combine the advantages of each material.
Currently, the scientific world is greatly interested in perovskite, a material used in photovoltaic applications since 2012 with unbelievable efficiency. In your opinion, could this be a new turning point for solar energy?
P. R. C.: Yes, the flavor of the moment is indeed the perovskite cell, a type of hybrid solar cell comprising a mixture of organic and inorganic materials. Yet many areas still require elucidation in this sector, particularly regarding cell stability, for example at high temperatures. But for the moment, perovskite cells have still not proven their worth at the industrial scale.
D. L.: They represent a revolution in the field. Even if the issues of stability and reproducibility have not been resolved for the time being, perovskite cells open up a new horizon for photovoltaic energy production. At the moment, there is a great interest for combining these perovskite cells with existing cells that use silicon or CIGS. These so-called tandems (stacking 2 elementary cells) could in theory achieve efficiency of more than 40%. For this reason, one of the key projects at the Institut Photovoltaïque d'Ile-de-France (IPVF), where we both work, is devoted to the investigation of tandem cells.
Have any research pathways been abandoned over time?
D. L.: Yes, a number of sectors have virtually disappeared. A few years ago, for example, there were high hopes for copper sulfide, since the constituting elements are abundant in nature, non-toxic and presents remarkable light-absorbing properties. In the 1980s, people thought it was the Holy Grail. However, the resulting cells were not sufficiently stable and deteriorated after a few days.
P. R. C.: There are other areas in difficulty, like organic photovoltaic cells. There was a boom in these materials in the 2000s, but it was very short-lived. Many researchers working in this branch abandoned these cells in favor of perovskite cells. While some organic cells unquestionably still have stability, efficiency and cost issues, the sector cannot be dismissed since it remains attractive in certain highly-specific markets.
Are recycling and the life cycle of photovoltaic cells important considerations in solar research?
D. L.: These factors are extremely important. We analyze all aspects of the life cycle of cells, from mining through to recycling. We are looking for life time of 20 to 30 years or more. Solar panels might even be passed on as heirlooms from one generation to the next!
P. R. C.: The recycling market is developing extremely well. The Energy Pay Back Time (EPBT), is only one or two years. In other words, within this time, a cell will have produced the same amount of energy as that required for its manufacture. The energy will have been “reimbursed” and the 20 or 30 years of remaining cell life may be seen as a bonus.
You both work for the Institut Photovoltaïque d'Ile-de-France (IPVF), which employs over 200 researchers working in solar energy. What are the ambitions of this institute for the future?
P. R. C.: France is a world leader in research on photovoltaic solar energy, but much work goes unseen. The IPVF provides a showcase. It also brings together a number of different sectors such as thin-films , silicon and perovskite cells, amongst others.
D. L.: The creation of IPVF enables France to build for the future. We feel that the key decade will be 2020- 2030. These will be the solar power years and by that time, the estimated capacity for production of solar electricity will exceed one terawatt! The key “30/30/30” objective of the IPVF is to achieve 30% efficiency at $0.30 per Watt by 2030. To this end, our establishment offers remarkable synergy between the public and private sectors, bringing together academic partners such as the CNRS and the École Polytechnique, major industrial companies such as EDF, Total and Air Liquide, but also smaller companies like Horiba Jobin Yvon and Riber. The CNRS is at the heart of this project, something we are very proud of—but it also means a great responsibility.
Today, solar energy represents only 1% of the energy produced worldwide. Long term, will it be able to replace fossil fuels?
P. R. C.: Of course. Coal may appear cheaper, but that is because all of the long-term costs with regard to health and the environment are not factored in. Solar energy is already very competitive in many regions of the world, such as Arizona (US) for instance. The future is not in fossil fuels, even if the switch is obviously not set to occur in the next couple of years. Solar energy can be used for anything from charging a mobile phone battery to supplying the energy requirements of an entire village, and is highly modular. And solar energy constitutes an unlimited and cheap resource.
D. L.: In the next 20 years, it is very likely that solar energy may overpass the standard sources of energy supply for electricity production. Today in some countries, almost 10% of electricity consumption derives from photovoltaic sources. We will learn to adapt our energy production methods according to the seasons, favoring solar energy in summer for instance and wind or hydraulic energy during the winter months. In the end, the system will balance itself out. Those who work in solar energy are naturally motivated by the idea that it represents a benefit for humanity.
Related topics:
Capturing the Sun in a Cell
Solar Energy Aims for the Sky
Photovoltaics: the Perovskite Rush
- 1. Director of the Institut de Recherche et Développement sur l'Énergie Photovoltaïque (IRDEP), joint lab between CNRS, EDF and Chimie ParisTech PSL, and Scientific Director of the IPVF.
- 2. Director of the Laboratoire de Physique des Interfaces et des Couches Minces (LPICM), CNRS-Ecole Polytechnique and Director of the Fédération de Recherche sur l'Énergie Photovoltaïque (Fed PV).
- 3. Copper gallium indium selenide (CIGS), for which the highest recorded efficiency is 22.6% in thin-layer cells.
Explore more
Author
Léa Galanopoulo has a biology degree and is currently studying scientific journalism at Paris-Diderot university.