You are here
The James Webb Space Telescope is set to explore the early Universe
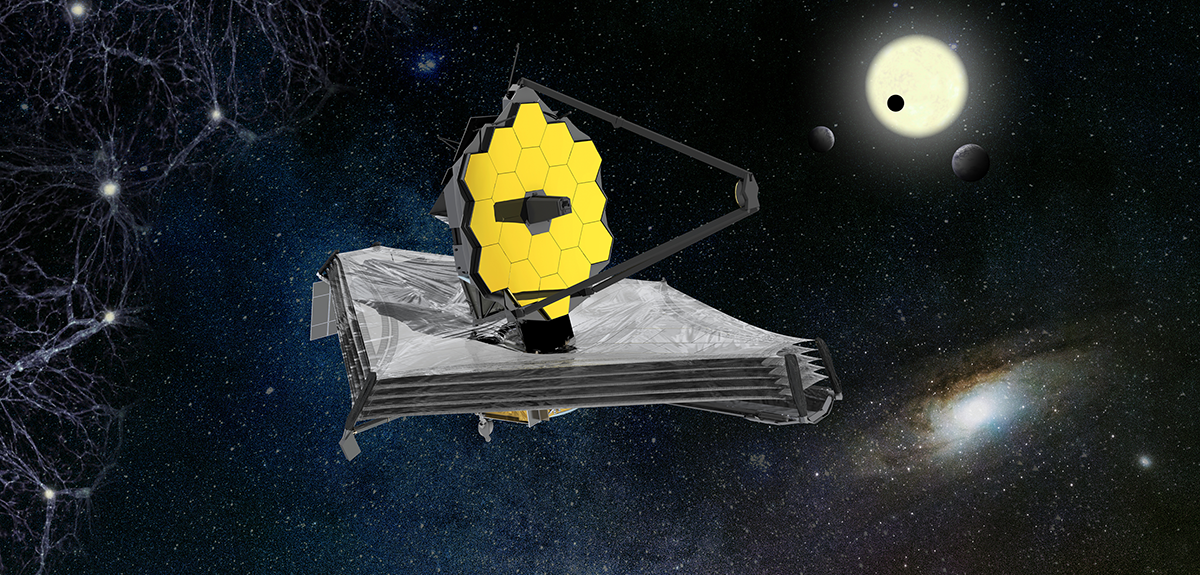
Claimed to be a hundred times more powerful than Hubble, which has been operational for the past thirty-one years, and equipped with a mirror 6.50 metres in diameter, NASA's James Webb Space Telescope (JWST) will be the world's largest orbital observatory. To say that the telescope, scheduled for launch on 18 December, is eagerly awaited by the world astronomy community would be an understatement. The preparatory work turned out to be fraught with difficulties: the instrument is so large that it took considerable engineering ingenuity to fold it up, together with its eighteen hexagonal mirrors, so that it fits into the Ariane V launcher's payload fairing. However, all this effort should prove well worthwhile: the JWST will provide astronomers with unprecedented possibilities for the observation of galaxies that are both distant and ancient, as well as planetary systems in our own Milky Way. Several CNRS teams are involved in this NASA programme.
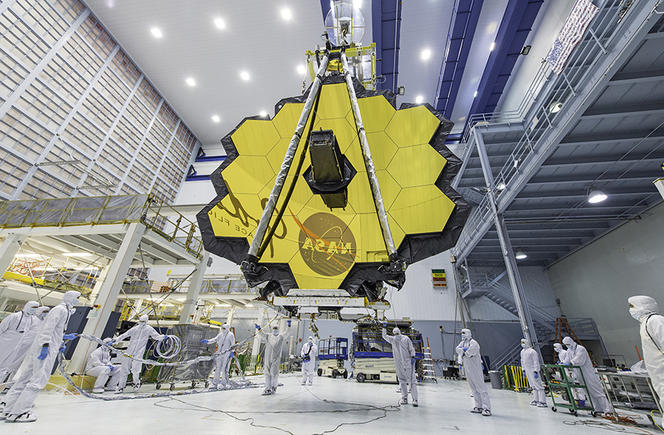
Seeing further with infrared
"The JWST fills a gap between Hubble, which only ventures into the near infrared, and the European Space Agency (ESA)'s space telescopes Herschel and ISO1 which go deep into the far infrared," says Daniel Rouan, research professor emeritus at the LESIA 2. "This new instrument will make it possible to observe at wavelengths ranging from around 0.5 to 30 micrometres, providing hugely increased sensitivity. This will enable us to see relatively cold objects, such as the dust and interstellar gas in galaxies (including our own), as well as planets and very distant galaxies. Although these are not cold, their energy emissions exhibit an apparent shift towards the infrared due to the expansion of the Universe."
Certain molecules are also easier to detect at these frequencies. For instance, hydrogen, the main component of the interstellar medium, is almost invisible outside these infrared band emissions, unless it is heated to very high temperatures. As for ground-based observations, infrared radiation from space is unfortunately massively absorbed by the atmosphere, and at wavelengths between 10 and 800 micrometres is even completely absent. In fact, it is this absorption that is responsible for the greenhouse effect caused by gases such as carbon dioxide and water vapour. The little remaining infrared radiation that does get through suffers from interference by a host of more powerful sources, both natural and artificial.
Although telescopes have been installed in high-altitude observatories, balloons and aircraft to try to mitigate these problems, satisfactory detection of faint energy sources in the sky has proved impossible. And it shouldn't be forgotten that the observation equipment itself emits infrared radiation.

Placed in an orbit parallel to that of our planet, at a distance of some 1.5 million kilometres (four times the distance from the Earth to the Moon), the JWST will avoid these drawbacks. Moreover, this environment will help the telescope to maintain a temperature low enough to ensure that its own thermal infrared emissions do not interfere with the radiation emitted by the very faintest objects.
Probing galactic cores
Once the JWST is in position and properly deployed, it will be able to start using its four instruments. NIRCam, NIRSpec and Niriss are respectively a camera, a multi-object spectrometer and a wide-field spectrometer, all designed to operate in the near infrared. MIRI3 is a camera and spectrometer operating in the mid-infrared range at wavelengths of 5 to 28 micrometres. It was developed in Europe, with the French Alternative Energies and Atomic Energy Commission (CEA) playing a major part. "MIRI has a key role because it covers a unique range of wavelengths that is of crucial importance for astrophysics. The instrument is very versatile, carrying out imaging as well as spectroscopy that can detect highly specific spectral lines," Rouan explains. The researcher, who has been working on the project for twenty-one years, hopes to probe galactic cores that contain a supermassive black hole.

He is also planning to observe exoplanets, which are very difficult to image directly because they are drowned out by the light from their stars, which is between a hundred thousand and ten billion times brighter. To see them, it is necessary to use optical devices called coronographs, which mask most of the light from the star. Rouan in fact invented the principle underpinning three of MIRI's four coronographs. NIRCam, made in the US, is in some ways similar to MIRI, but targets wavelengths of less than five micrometres. NIRSpec, mainly built by industrial partners from Europe, can obtain the spectra of several objects at a time, whereas MIRI studies them one by one, focusing on smaller regions, and in various spectral regimes. NIRISS was designed in Canada and combines the capabilities of the other three, while retaining enough specific features so as not to be redundant.
Understanding the formation of the Solar System
All this technology gives rise to fierce competition for observation slots. The teams who helped to produce the JWST's instruments benefit from guaranteed observation time. The others have to respond to calls for tender, which is how the project put forward by Olivier Berné, a CNRS researcher at the IRAP,4 was selected from among some one hundred proposals. "We are focusing on the Orion Nebula, because the current scientific consensus is that its environment is similar to that of the formation of our own Solar System, in the vicinity of particularly massive stars," Berné explains.
Observing it would therefore be like going back in time to these primordial conditions, enabling scientists to study the composition of the gas and dust in molecular clouds before they collapse. It is this process that leads to the formation of a star and a disc, from which in turn planets, and perhaps life, are born. "We wish to understand the initial ingredients required to bring about the chemical processes that make this happen," the researcher explains.
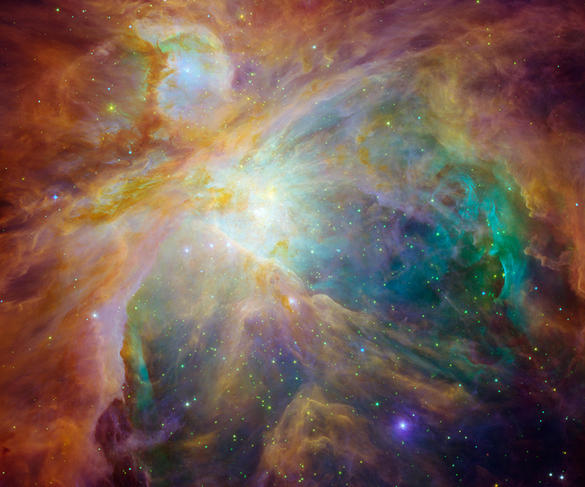
The JWST will also be used to investigate a phase dating back to 7 billion years after the Big Bang, when the Universe was half its current age. "We pick up signals from galaxies from that time, but we can't see what's inside them. Studying Orion could provide a toolbox to help specialists of that period interpret them," Berné points out.
In September 2022, he and his colleagues will have 35 hours of observation time in which to obtain as much data as possible, which the researchers hope will enable them to find answers to both these questions. "We have been preparing for this since 2014, using simulations and ground-based observations," Berné says. "When our turn comes, we will have to ensure that all the settings are fully optimised to collect the best possible data, rather as a professional photographer would. The difference is that, to capture as many photons as possible, we'll have to calibrate with hair's breadth accuracy a telescope weighing more than six tonnes and located 1.5 million kilometres away. But we are going to gain so much detail in return that, as I like to put it, we'll be moving from impressionism to Dutch realism!"
Going back in time to the first stars
Berné is not the only one eagerly awaiting the launch of the telescope. Nicole Nesvadba, a CNRS researcher at the J-L Lagrange Laboratory5 in charge of the JWST's "General Observer" programme, has obtained three slots, spread over the five years during which MIRI should be operational. "We are working on supermassive black holes in galaxies. These are located at the centre of quasars, which are highly luminous active galactic nuclei," Nesvadba explains. "These objects may determine the formation of stars and the increase in mass of the largest galaxies that host them, and we want to understand how. With the JWST we will be able to observe these processes throughout most of the history of the Universe, right back to the very first of these black holes."
Nesvadba also mentions the phase of rapid, sustained star formation two to three million years after the Big Bang, which was followed by a sudden drop in the birth of new stars six billion years later. "We don't yet understand what caused such a slowdown when there was still plenty of gas available for the process to continue," she admits. "One possibility we're looking at are the interactions between black holes, quasars and galaxy formation."
The energy injected by black holes into the gas clouds surrounding them may play an important role. The JWST will provide a unique opportunity to observe this phenomenon in galaxies relatively close to our own, and on various scales. The researchers are therefore anxious to see the JWST in operation, and need to get ready for the arrival of the data.
The project has been seriously affected by delays, and some teams have been waiting to resume the mission for years. "The initial schedule provided for a launch in 2008," Rouan points out. "Some industrial partners in the US came up with rather 'odd' cost estimates, and Congress almost shut down the whole project in response. However, all the instruments under the responsibility of industrial and academic teams in Europe were delivered on time." More than twenty years after the beginning of the project, the James Webb Space Telescope is finally ready to become a key player in space observation.
Author
A graduate from the School of Journalism in Lille, Martin Koppe has worked for a number of publications including Dossiers d’archéologie, Science et Vie Junior and La Recherche, as well the website Maxisciences.com. He also holds degrees in art history, archaeometry, and epistemology.