You are here
How to Deflect a Killer Asteroid
Reading time: 16 minutes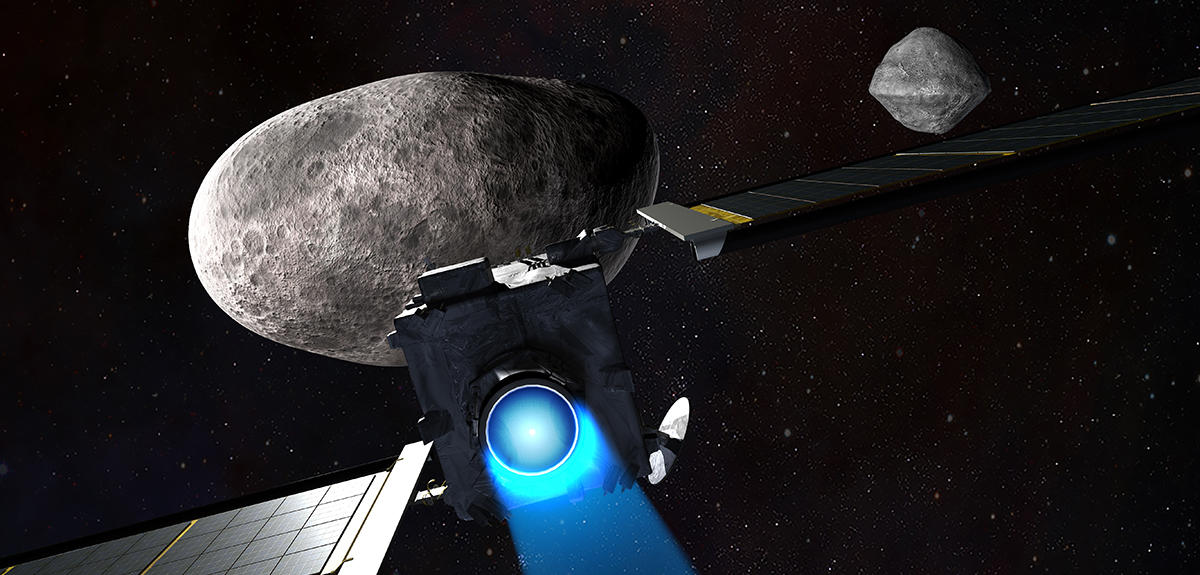
In November 2019, the Ministerial Council of the European Space Agency (ESA) gave the go-ahead for the launch of the Hera mission, for which you are the scientific coordinator. Can you outline the history of this European research project, which has been postponed a number of times?
Patrick Michel: The idea of the project first arose in 2004, when the ESA asked a group of six scientists, including myself, to plan a series of missions aimed at addressing the risk of an asteroid impacting the Earth. Of the six proposals, two were designed to approach such an object as closely as possible in order to characterise its structure using radar or microsatellites. Another of the missions, known as Don Quijote, featured an asteroid deflection trial. At the time, this combined an impactor, called Hidalgo, and an orbiter named Sancho, whose job was to measure the effect of the deflection. Due to lack of funding, Don Quijote was shelved for a few years, before re-emerging as a collaboration, now renamed AIDA, between NASA and the ESA.1
Although in 2016 the European component of the project, initially christened AIM, was unable to obtain the funding it needed from the ESA Ministerial Council, it was nonetheless welcomed by the international community working on small bodies and planetary defence. Encouraged by this support, we therefore conducted another study aimed at optimising the project, now dubbed Hera, before successfully resubmitting it to the Ministerial Council in 2019. So it took us fifteen years to finally make this mission a reality.
Now that the whole AIDA project has been confirmed, what is the main purpose of this international research programme?
P.M.: In its current configuration, the project combines the DART impactor, designed by the US, and the Hera orbiter, which will measure the deflection of the asteroid after the collision. Hera will also carry out a detailed characterisation of the asteroid’s physical properties and composition. The mission will target the binary asteroid Didymos, which is made up of a principal body and a smaller one in orbit around it, informally named Didymoon, which will be impacted by DART.
Targeting a binary body will enable us to instantaneously observe the effects of the deflection, since Didymoon orbits around the main asteroid very slowly in comparison with DART’s impact velocity. In addition, Didymos will pass relatively close to the Earth at the moment of impact, which opens up the possibility of using Earth-based observatories to measure the change in Didymoon’s orbital period around the main asteroid. Although the goal of the DART/Hera mission focuses on planetary defence strategies, the data collected will also be of great interest not only to scientists who study small celestial objects but also to those concerned with potentially exploitable resources in space.
How will Hera fit in with the American part of the mission?
P.M.: The US satellite will launch from California in July 2021 and will reach Didymos in October 2022. Before DART hits the smaller asteroid, Didymoon, the mission will deploy the LICIA CubeSat. This mini-satellite developed by the Italian space agency will be used to view the first seconds of the impact. However, it won’t be able to observe the formation of the crater due to the cloud of dust produced by the collision. At the same time, an international observation campaign using Earth-based radars and optical telescopes will measure the variation in the orbital period of Didymoon caused by the shock. Hera will then take off from French Guiana in 2024, reaching the asteroid in January 2027. The spacecraft will subsequently make a detailed analysis of the effects of the impact.
In what way is this project not only unprecedented but also key to designing an effective planetary defence system?
P.M.: Firstly, Hera will be the first space mission to rendezvous with a binary asteroid, a configuration that makes up 15% of the asteroids that enter the Earth’s neighbourhood. With a diameter of barely 160 metres, Didymos’s moon, Didymoon, will be the smallest such body ever visited. The mission will also be the first to probe an asteroid’s interior: one of the CubeSats accompanying Hera will be equipped with a radar able to analyse Didymoon’s deep structure. Within the Hera team, the scientific expertise for this technique, in which France is a leading player, will be provided by Alain Hérique, a planetary scientist at the IPAG.2

As regards planetary defence, Hera will calculate Didymoon’s mass as well as the properties of the impact crater. Measuring the mass is key to quantifying the momentum transferredFermerQuantity equal to the product of the mass and velocity of a moving body. When two objects collide, the total momentum of both bodies is conserved. by the DART projectile when it hits the asteroid. If no matter is ejected during the impact, only DART’s momentum will be transferred, and the deflection will therefore be minimal. Conversely, if a large amount of matter is released, the deflection will be maximised. To determine the result, we will need to know Didymoon’s momentum. This depends on its mass, which only Hera will be able to measure.
In addition, the amount of matter expelled is also a function of Didymoon’s internal structure. For example, a porous body will absorb the collision, which will reduce the amount of ejected matter and thus the deflection produced. In order to fully assess the effects of the impact, we must ascertain the momentum transferred by the shock, the properties of the crater, and Didymoon’s structure. This information will also enable us to validate the deflection technique as well as the numerical impact simulations so that they can be applied to other scenarios with a higher confidence level. Without this direct data obtained in a real-life situation, we will not be in a position to make any progress in this area.
What significance does this mission have from the point of view of fundamental research?
P.M.: Collisions have been crucial in the formation and evolution of our Solar System. The planets were initially formed by slow-moving shocks in which large amounts of material stuck together. In a second stage, giant impacts gave rise to natural satellites such as the Moon. Today, we are at a stage when asteroid crashes are breaking them up into smaller fragments or are producing craters on every celestial body in the Solar System. Understanding these processes, on scales that are impossible to achieve in the laboratory, will allow us to better define and characterise the history of collisions in our Solar System.
By giving us the opportunity to study a large-scale impact of which DART will record the initial context, and Hera will measure the final conditions, the mission will make it possible to take a giant step forward in our knowledge of crater formation processes in the Solar System. Moreover, to improve our understanding of small bodies, we need to elucidate the mechanisms governing them under their very low-gravity conditions. This fascinating challenge is of interest to a wide range of scientific communities, including those that study the dynamics of granular environments.
As a researcher, you have a special interest in the origins of near-Earth objects (NEOs). What were your scientific contributions in this field?
P.M.: My work helped to show that most of these objects, which are less than a few tens of kilometres in size, result from the clumping together of fragments from collisions between asteroids in the main asteroid belt, located between the orbits of Mars and Jupiter.3 Such aggregates of rocks are sometimes found in unstable regions, which can cause them to shift from circular orbits to more elongated ones where there is a possibility that they cross the Earth’s orbit. Over the past four billion years this population of celestial bodies has remained stable, since its ‘mortality rate’, mainly caused by the Sun’s attraction, is constantly offset by the formation of new objects in the belt.
By analysing the impact craters on the Moon and theoretical models of NEO populations, including those developed in our laboratory, we reckon that there are about 1,000 NEOs with diameters exceeding 1 km, 90% of which have already been identified (which was the goal set for NASA by the US Congress in 1998). Our models have also shed new light on the NEO population. A paper published at the beginning of the year in the journal Icarus4 has drawn up an almost complete inventory of these objects in terms of size, distribution and albedo (reflectivity), all of which tells us about their composition.
How did we first become aware that collisions with celestial objects could be a threat to life on Earth?
P.M.: Until the late 1960s and the Apollo programme’s first lunar exploration missions, the scientific community was convinced that the craters seen on the surface of the Moon were of volcanic origin. Analysis of the samples collected during the Apollo missions finally showed that these were in fact caused by meteorite collisions. One of the very first political acts that acknowledged the hazard associated with the impact of an asteroid was a European resolution adopted in 1996. This inititative was prompted by comet Shoemaker-Levy colliding with Jupiter in July 1994, the first event of this kind to be directly witnessed by humankind.

At an international workshop on NEOs held in Turin (Italy) in 1999, the community of experts on small bodies subsequently set up the well-known Torino Scale. This system, which was meant to be understood by the general public and the media, aimed to provide a measurement of the impact hazard associated with any asteroids threatening the Earth. At the same time, an increasing number of NEOs were discovered, and the initial calculations of their paths, obtained from their estimated orbits (which are still very uncertain when they are carried out on the basis of early observations), sometimes resulted in a non-zero probability of collision with the Earth over shorter or longer time scales.
The discovery in December 2004 of an NEO called Apophis was another turning point in this growing awareness.
P.M.: Trajectory calculations based on the initial observations of the object, which has a diameter of 325 metres, effectively gave a very high chance of collision with the Earth in 2029. Although new radar investigations carried out in 2013 subsequently showed that the asteroid would merely brush by our planet in 2029 and then again in 2036, we suddenly realised that there was no protocol to respond to a disaster of this nature. With a view to setting up an international decision-making process on these issues, I took part in the work of a team commissioned by the UN. This led to the creation of two separate working groups: the first, made up of asteroid specialists, was tasked with predicting the chances of an impact with one of these celestial bodies, while the second brought together the various space agencies in order to organise a possible response to the hazard.
Since 2009, international space agencies and scientific experts have also been meeting at the biennial Planetary Defense Conference to present advances in impact simulation studies, as well as various ideas for missions aimed at deflecting asteroids. On these occasions, a virtual exercise based on a scenario developed by colleagues from the Jet Propulsion Laboratory (California, US) enables us to test our ability to respond to this risk. Since 2012, the European Commission has also been funding consortia — such as NEO-MAPP, of which I am the coordinator — to study this problem.
How can the astrophysics community help predict this type of disaster, which after all remains exceptional?
P.M.: Admittedly, this is the least likely of natural disasters, in comparison with other hazards such as earthquakes, tornadoes and tsunamis. But when one does occur it could have very serious consequences. Unlike earthquakes, we now have the means to predict and prevent an asteroid strike.
To do this, two courses of action must be taken. First, we need to make the most exhaustive inventory possible of objects that exceed 140 metres in diameter, which is the threshold above which an asteroid will affect inhabited areas in whatever region of the world it strikes. In a few decades, it should be possible to achieve this from Earth, using next-generation ground-based telescopes such as the LSST in Chile and Pan-STARRS on the Hawaiian island of Maui. However, within a decade, the American NEOSM mission, which has received encouraging support from the US Congress in its 2020 budget, should be able to do this from space, starting in 2025. Once we’ve completed this catalogue, we should be in a position to determine whether any of these objects pose a direct threat. If, in parallel with this survey, the first attempt at deflecting an asteroid carried out by DART/Hera proves successful, we will have gone a long way towards solving the risk of asteroid collisions.
You are also closely involved in the Hayabusa2 programme, overseen by the Japan Aerospace Exploration Agency (JAXA), and in OSIRIS-REx, funded by NASA. The aim of both these missions is to bring back to Earth samples collected from the near-Earth asteroids Ryugu and Bennu, respectively. Have these two missions yet enabled scientists to find out more about such near-Earth asteroids?
P.M.: Apart from information such as their shape and size, we knew nothing so far about the structure and composition of these objects or about their surface features. All the scientists who, like me, have been lucky enough to take part in these missions have had an extraordinary opportunity to explore two asteroids that were completely uncharted territory located several hundred million kilometres from Earth. Among our most surprising discoveries were the abundance of boulders and the lack of any rock-free areas larger than five metres in diameter.

On one and the same body we also observed extremely varied rock morphologies, whereas we had expected to find a relatively uniform environment. In January 2019, the OSIRIS-REx programme also showed that Bennu was an active object with particles escaping from its surface. A paper published last year in the journal Science5 outlines the different scenarios that could explain this plume of particles, which remains something of a mystery. As well as scientific advances, these missions to small celestial objects are able to capture the general public’s imagination, due to the atmosphere of suspense that surrounds them. This is confirmed by the success of the talks that I give, which aim to get the excitement of these challenging missions across to younger people.
What are we likely to learn from the analysis of the samples that will have returned to Earth by the end of the year for Hayabusa2 and by 2023 for OSIRIS-REx?
P.M.: First of all, we should be able to determine the properties of the basic building blocks present in the early Solar System that gave rise to the formation of the planets. In the various scenarios developed at the J.L. Lagrange laboratory in Nice , we have already shown that a massive asteroid bombardment occurred towards the end of the Earth's formation, around 4.7 billion years ago.
We still don’t know whether the prebiotic material that led to the emergence of life on our planet came from the asteroids. Once we have collected organic materials from the samples returned from Bennu and Ryugu and subjected them to a high-precision analysis in research laboratories, we may be able to find out whether life on Earth was the result of bombardment by meteorites. Measuring the degree of shock in these same samples should also tell us about the intensity of the collisions experienced at the time these asteroids were formed and during the early stages of our Solar System’s history.
To what extent will the findings of missions designed to study near-Earth asteroids help to anticipate their possible impact with the Earth, while implementing effective strategies to prevent such a disaster?
P.M.: By studying NEOs the missions will improve our understanding of these potential enemies, even though personally I prefer to think of them as friends! Thanks to Hayabusa2 and OSIRIS-REx we now know for example that an asteroid with a diameter exceeding 400 metres can have a large number of sizeable rocks evenly distributed over its surface. This characteristic is therefore now taken into consideration when drawing up deflection strategies.
We have also discovered that the density of dark carbonaceous objects like Bennu and Ryugu scarcely exceeds that of water, since these bodies are very porous. So in the event of an impact, a large amount of the energy would be dissipated by the compaction of the porous parts. We therefore believe that the force required to deflect an object of this kind would have to be greater than for a brighter, denser stony asteroid such as Itokawa, investigated in 2005 by the Hayabusa1 mission, and samples of which were brought back to Earth in 2010. However, all this is still uncertain, and the result of DART’s impact is eagerly awaited in order to verify this hypothesis.
Does this mean that the planned defence system will depend not only on the size of the object involved but also on its composition and internal structure?
P.M.: A priori, all this data will have to be taken into consideration before an asteroid is deflected. If the NEOSM mission comes to fruition, it will be possible to characterise the size and density of a potentially threatening object at a very early stage, which will then enable us to calculate the amount of energy needed to deflect it.
That said, the deflection test carried out by the DART/Hera mission will be performed blind, since we have no prior information about the structure and composition of Didymoon. If this first attempt is successful despite the uncertainties, we will then know that the precise nature of the target asteroid is not necessarily a determining factor when it comes to deflecting such an object. Yet in the absence of this real-life test, we are for now completely in the dark.
- 1. Senior researcher at the CNRS, head of the Planetary Science group at the J.L. Lagrange laboratory in Nice (Unité CNRS / Université Côte d’Azur / Observatoire de la Côte d'Azur).
- 2. Institut de planétologie et d'astrophysique de Grenoble (CNRS / Université Grenoble-Alpes).
- 3. “Collision and gravitational reaccumulation: forming asteroid families and satellites”, P. Michel et al., Science, 2001, vol. 294 :1696-1700. https://doi.org/10.1126/science.1065189
- 4. “Debiased albedo distribution for Near Earth Objects”, A. Morbidelli, M. Delbo et al, Icarus, 11 January 2020. On line
- 5. “Episodes of particle ejection from the surface of the active asteroid (101955) Bennu”, D. S. Lauretta, C. W. Hergenrother et al., Science, published 6 December 2019. https://doi.org/10.1126/science.aay3544
Explore more
Author
After first studying biology, Grégory Fléchet graduated with a master of science journalism. His areas of interest include ecology, the environment and health. From Saint-Etienne, he moved to Paris in 2007, where he now works as a freelance journalist.