You are here
How optogenetics has restored partial sight to a blind patient
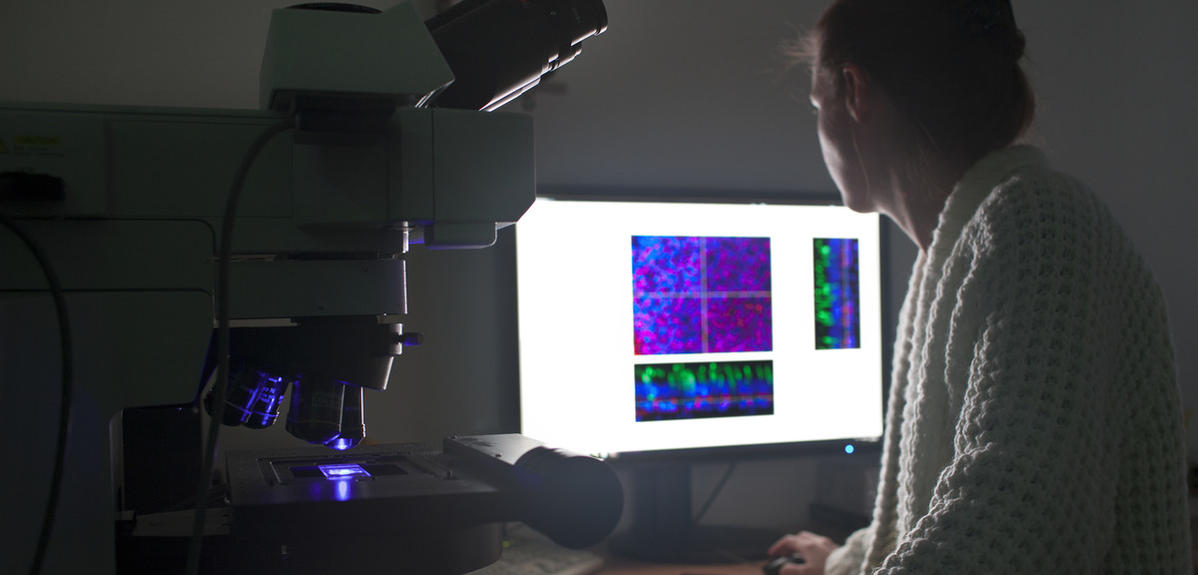
This medical and scientific feat was made possible thanks to optogenetics. Could you please explain?
José-Alain Sahel:1 Optogenetics consists in genetically modifying a cell – generally using a virus – so that it produces a photosensitive protein, which generates an electrical signal when it receives light stimulation in the corresponding wavelength. The discipline was born in the 1990s and early 2000s, when scientists initially focused on determining, from a biophysical point of view, the mechanisms underlying interactions between light and certain algae or bacteria. A German team from the Max Planck Institute identified a natural protein called channelrhodopsin, which can change the electric potential of a cell through its membrane as a function of light.
This was originally basic research work, but the scientists gradually realised that it might be possible to manipulate the electrical activity of cells, and particularly neurons. Neuroscientists thus took up the baton in order to understand the connections between networks of neurons in the brain. Valentina Emiliani, who has since joined the Institut de la Vision and was awarded this year’s CNRS Silver Medal, is using these findings to study networks in the cortex, while Claire Wyart, at the Brain and Spine Institute (ICM),2 applies them to her research on the zebrafish.

The first patent already mentioned the possibility of clinical applications, and an increasing number of laboratories started to take an interest in optogenetics. It was even referred to as the “Method of the Year 2010” by Nature Methods, and according to Science is one of the main breakthroughs of the decade!
I would like to point out that we started from basic research on proteins in algae and the processing of information by the nervous system. And yet, we have managed to achieve the very first clinical application of optogenetics, which shows that the distinction so often made between basic and “applied” research is totally misleading.
How did you decide to apply optogenetics to the treatment of blindness?
J.-A. S.: In 2007, I started discussing the issue with Botond Roska, now co-director of the Institute of Molecular and Clinical Ophthalmology Basel (IOB) (Switzerland), who had applied this technique to the study of circuits in the retina. The idea of using response to light to induce the production of an electrical current made us think about what was happening in the retina. We therefore decided to try and apply optogenetics to visual impairment. Although some of our colleagues thought this project was ludicrous, we held our course because we knew it made sense.
The first trials, conducted in Detroit (US) by another team, used a protein that required very strong light – only too strong for clinical applications. Despite an excellent publication, the studies do not seem to have been a success. As for us, we were able to identify three major challenges that needed to be addressed to achieve clinical applications: choosing the right proteins for the right type of cell, determining how they could be stimulated effectively, and developing visual rehabilitation protocols for patients.
This project required a lot of time and a great deal of tenacity. It was difficult to find funding over these twelve years, and we had to hold our nerve when the trials were unsuccessful. But we never gave up.
How did you choose which protein to be injected and the type of cell to be targeted?
J.-A. S. Botond Roska initially explored bipolar cells in the retina, which split the sensory signal into on and off. However, in primates these neurons proved too complicated to target for the injection of a gene coding a protein. A group of Swiss scientists is still working on this option, but at an earlier stage.
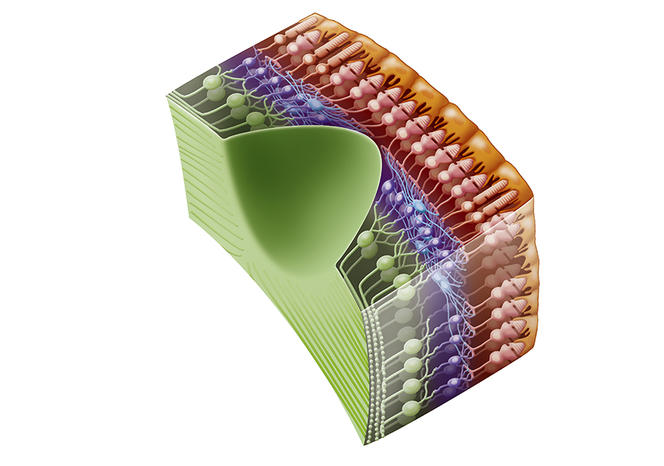
We then turned to dormant photoreceptors. In diseases such as pigmentary retinopathy, the cells gradually lose their photoreceptive properties but they do not die and the connections between them are still in place. We succeeded in reactivating these cones and in 2010, with Botond Roska and Serge Picaud, published our findings in Science, which enabled us to obtain financial backing from the Foundation Fighting Blindness. However, we were not able to express the correct proteins sufficiently strongly in these cells. Botond Roska in Basel, and Deniz Dalkara at the Institut de la Vision, are still exploring this avenue using different approaches, and it might re-emerge in a few years’ time.
Our attention then turned to ganglion cells in the optic nerve. Although they are not photoreceptive, they react naturally to light because they contain melanopsin, a photopigment that regulates the day/night cycles of the body. However, this pigment responds too slowly for a strategy to restore vision, so it was necessary to determine which protein should be expressed in these ganglion cells, knowing that we had to eliminate those that required a high level of light, which would cause damage.
Ed Boyden, Professor at the Massachusetts Institute of Technology (MIT), works on modifying natural proteins in order to sensitise them to a specific type of light. This issue is similar to that addressed by Roger Tsien, who received the Nobel Prize for Chemistry in 2008 for his research on green fluorescent protein. While we were testing Tsien’s GFP, Boyden suggested that we should try the Crimson R protein that reacts to low-energy amber light, and indeed we were able to target it effectively to the membrane of ganglion cells. The natural version of this protein comes from algae that use it to orient themselves thanks to light from the water’s surface.
How do you manage to stimulate this protein?
J.-A. S.: We needed a projector that was able to send this amber light into the eye. The protein requires not only a precise wavelength but also a constant level of brightness, and these parameters vary considerably in ambient light. A team from GenSight, working with the Institut de la Vision, therefore developed bio-inspired cameras that can detect these changes, pixel by pixel and to the nearest microsecond.


These cameras were mounted on glasses that code this signal and then project it into the eye at the right wavelength. Because the design of this system was very expensive, and traditional pharmaceutical corporations tend to focus on molecular methods and have no expertise in medical devices, we set up a company called GenSight Biologics with Bernard Gilly, Serge Picaud and Botond Roska. This start-up has consequently developed both the gene therapy and the equipment necessary for its application.
Once all these elements are combined, how can patients benefit?
J.-A. S.: In early 2019, we started to work with blind subjects, injecting vectors able to transport a gene coding for the Crimson R protein to the ganglion cells. The vectors were viruses modified to be non-pathogenic that could easily penetrate the retina and transmit the genetic code for Crimson R as efficiently as possible. The patient whose treatment is described in our publication in Nature Medicine received the injection at Hôpital des Quinze-Vingts in Paris; it was then necessary to wait several months for the protein to be sufficiently expressed in order to proceed.
The volunteers were then scheduled to start their rehabilitation with StreetLab, a platform that reproduces real-life conditions. It also enables us to study the movements of the bodies and eyes of patients once they have been trained to use the spectacles and carry out different tasks, as well as adapt the devices to their needs and comfort.


In February 2020, one individual announced that he had seen the white stripes of a street crossing. He then became able to identify, count and grasp various objects: a notebook, paperclips, a glass of water, etc. Our system does not restore normal vision, but offers an image with clear outlines that correspond to the shapes of different objects. In addition, working with Angelo Arleo at the Institut de la Vision, we clearly detected activation of the visual cortex in this patient, which corresponded to accurate visual decoding.
Unfortunately, the onset of the Covid pandemic disrupted our plans and the other six patients treated in Paris, London (UK) and Pittsburgh (US) were not able to visit us often enough to be sufficiently stimulated and become familiar with using the spectacles. However, seven people received the injection, performed in groups of three in successive stages. The dose of the injected vectors is increased gradually in order to make sure that it is not causing any problems. In the longer term, we hope to treat fifteen subjects.
Our research is carried out in close collaboration with the patients, who play an active role in our work. We are convinced that in terms of rehabilitation, close ties between actors, developers and users are essential. And indeed we are continuing to improve our spectacles.
Which type of blindness are these patients suffering from, and can your method be applied to all cases?
J.-A. S.: They are all suffering from terminal pigmentary retinopathy. However, this disease can be caused by more than 70 different genes whose defects converge to cause the same type of visual impairment. But the patients are not always affected by the same genetic disorder. We shall be pursuing our study and extending it to other types of degeneration, in other words to all retinal diseases that cause blindness and could potentially be treated using optogenetics; the only essential condition is that the optic nerve should still be functional.
What other conditions could optogenetics help to treat?
J.-A. S.: Optogenetic applications related to vision are one step ahead of the others, because the stimulation of neurons by light is a natural mechanism of the eye. Other fields are nevertheless concerned. Karl Deisseroth at Stanford University (US) is deciphering abnormalities of the neuronal circuits linked to psychiatric disorders, but there is no mention of clinical trials as yet. Tobias Moser at Göttingen University (Germany) is working on the auditory system and hopes to begin trials between now and 2025. Another German team intends to use optogenetics for deep brain stimulation in a bid to treat neurodegenerative conditions such as Parkinson’s disease.
What other work is being carried out at the Institut de la Vision, which you are heading?
J.-A. S.: Many of our members are studying the development of the visual system and its links to the nervous system. We work within a continuum between basic research and therapeutic or diagnostic applications. For example, we are looking into non-invasive cellular imaging, and the protection of central vision.
With a team from Stanford and the company Pixium Vision, we have developed the first wireless artificial retina in the world, and implanted it in several patients. Some of our scientists are exploring gene therapies that are not restricted to optogenetics, and the application of photoreceptor progenitor stem cells. Finally, we benefit from a platform to study the effects of ageing, visual impairments, and their management in terms of spatial orientation and vision.
Keywords
Share this article
Author
A graduate from the School of Journalism in Lille, Martin Koppe has worked for a number of publications including Dossiers d’archéologie, Science et Vie Junior and La Recherche, as well the website Maxisciences.com. He also holds degrees in art history, archaeometry, and epistemology.
Comments
Log in, join the CNRS News community