You are here
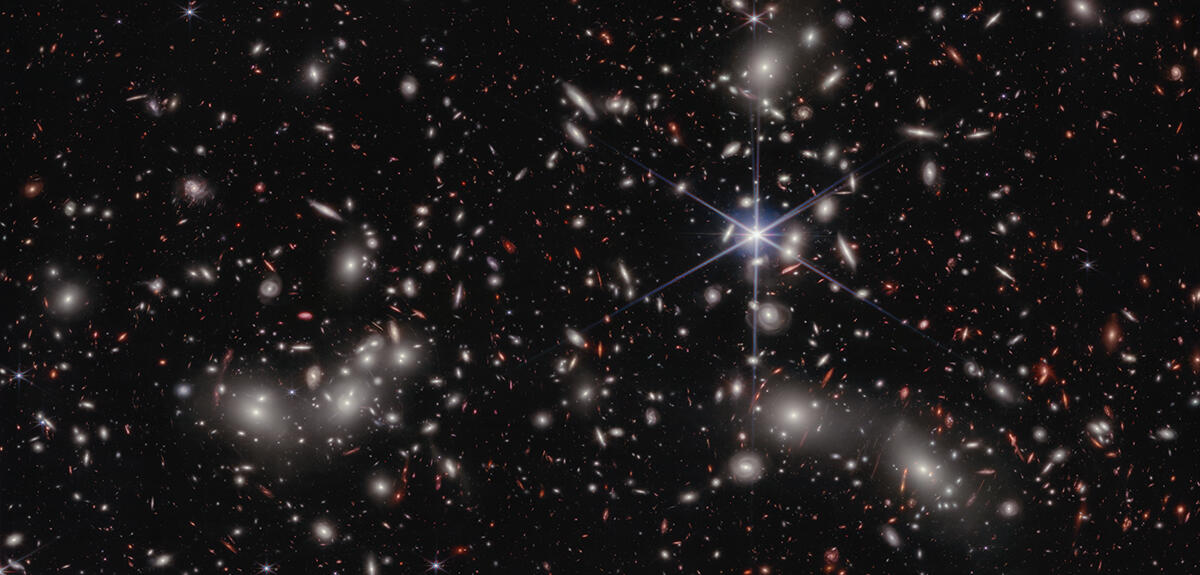
Cosmology is in crisis. Rather like a gigantic balloon, the Universe has been constantly expanding ever since the Big Bang, 13.8 billion years ago. The crux of the problem now shaking up the world of cosmology has to do with the rate at which this is happening. When astrophysicists use their telescopes to measure the current expansion rate, known as the Hubble constant (or H0), the value they come up with clashes with that predicted by the standard model of cosmology, the theory that so far best describes the history of the Universe from its inception (the Big Bang) and the very first atoms, all the way up to the present day, via the formation of the first stars and galaxies. So this is no trivial discrepancy: it could mean that this model, around which there has always been a consensus, needs to be totally reassessed. Unless of course there has been an error in the measurements. The question is being hotly debated among scientists, who are doing everything they can to resolve the “Hubble tension” as it has been dubbed.
Measurement methods
The American astronomer Edwin Hubble was the first to estimate the value of the constant that now bears his name. In 1929, he observed that galaxies were moving away from the Earth, and that the further away they were, the faster they were receding. The ratio of a galaxy's recession velocity to its distance gives H0. This discovery, which implied that the Universe was expanding, is the cornerstone of the Big Bang hypothesis, and marked the beginning of modern cosmology. Hence the importance for astronomers to measure the value of this parameter as precisely as possible. To make matters worse, astrophysicists have also known for the past twenty-five years that cosmic expansion is speeding up due to the effect of a mysterious dark energy, thought to make up two thirds of the Universe, and whose nature remains unknown. Ever since the earliest recorded observations, continuous improvements in techniques have led to significantly more precise measurements of the constant. Today, two principal methods are used to determine it.
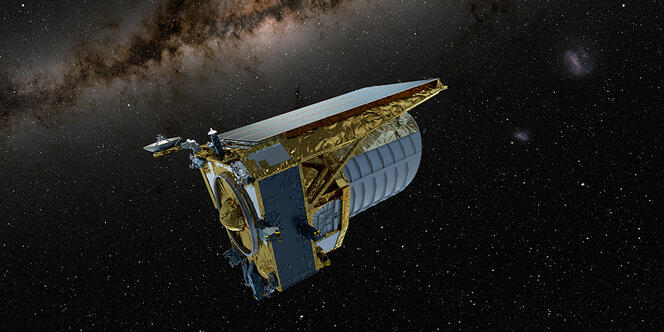
The first one is based on analysis of the cosmic microwave background, the very first light emitted by the Universe when it was just 380,000 years old. This radiation is still visible today and has been mapped in detail, in particular by Europe's Planck spacecraft. By reconstructing the conditions that prevailed in the primordial Universe, it proved possible to predict, within the framework of the cosmological model, the expansion rate that should be observed today. According to Planck data published in 2020, H0 has a value of 67.4 kilometres per second per megaparsec (Mpc) (one parsec is equal to 3.26 light-years). This means that over this range, the Universe expands by 67.4 km every second, and therefore that a galaxy at that distance is travelling away from us at that speed.
The second approach is to directly measure H0 by observing the galaxies around us. This means determining both the recession velocity and the distance of a large number of such objects, just as Hubble did in his time. The first step, calculating a galaxy's recession velocity, is straightforward, due to the redshift of its light spectrum: the faster the galaxy is travelling away from us, the greater its redshift.
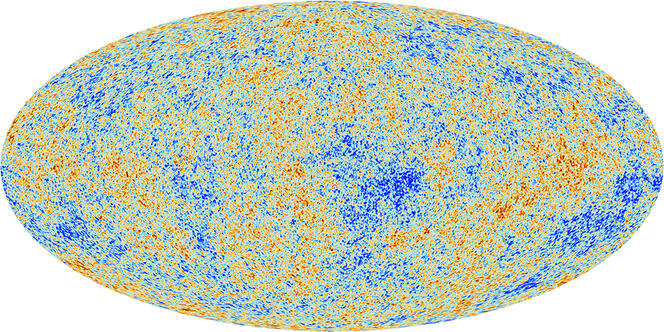
Standard candles and distance ladders
However, the second step, which consists in measuring the distance of a celestial body, is trickier. To do this, astronomers use what they call standard candles. “These are objects whose absolute luminosity i.e. their intrinsic brightness – editor’s note is known. By comparing this with their apparent brightness as seen from Earth, we can determine their distance and therefore that of the galaxy in which they are located,” explains Mickaël Rigault, of the Institute of Physics of the Two Infinities of Lyon (IP2I).1 One such standard candle are Cepheids, variable stars whose pulsation period is related to their absolute luminosity – a law established in 1908 by the American astronomer Henrietta Leavitt. This of course requires prior calibration of the intrinsic luminosity of a number of these stars by directly measuring their distance using a geometric method such as parallax. These were the celestial bodies that Hubble relied on to obtain an estimate of the cosmic expansion rate.
Today's astronomers resort to the same technique but have improved it by using a second type of standard candle, even further away than Cepheids, namely, what are known as ‘Type 1a’ supernovae, stars at the end of their lives that explode and for a few weeks become as bright as billions of suns. Just as with Cepheids, the precise luminosity of these supernovae has to be calibrated. This is done by using Cepheids in nearby galaxies where supernovae are also observed.
“In this way, we build up a kind of cosmic distance ladder where each of the rungs makes it possible to measure the distance of increasingly distant objects, thus enabling us to determine the Hubble constant with high precision,” Rigault explains. The latest measurement of H0, obtained by the SHoES collaboration led by the American scientist Adam Riess using this approach, yielded a value of 73 km/s/Mpc.
Inexplicable discrepancy and cosmic dust
At first glance, the discrepancy with the result from the analysis of the cosmic microwave background seems minor. However, when uncertainties are taken into account, the two estimates are incompatible. “Until a few years ago, there was still some doubt about the reality of this anomaly. However, measurements of H0 have now become so precise that the probability that this discrepancy is due to chance is less than one in a million. That’s very exciting, because it could mean that our model of cosmology is incomplete and that we need to consider the possibility of new physics,” says Vivian Poulin-Détolle, of the Montpellier Universe and Particles Laboratory (LUPM).2
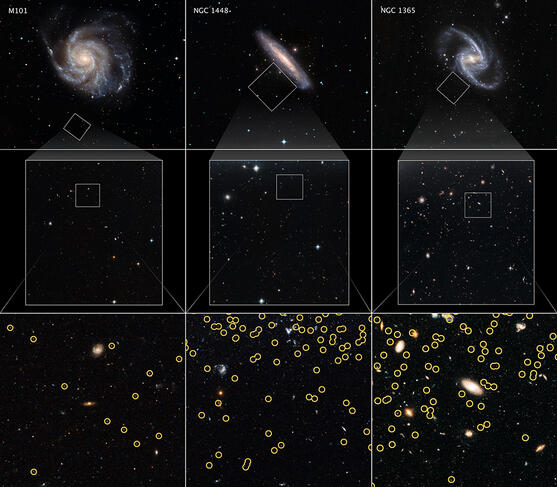
This observation is reinforced by the fact that other measurements obtained by independent methods arrive at a value of H0 consistent with that determined using Cepheids and supernovae. This is particularly true of the HOLiCOW experiment, which tracks distant quasars, celestial objects with very active and extremely luminous nuclei. When a large galaxy lies on the line of sight between us and a quasar, it deflects the light from the latter to such an extent that multiple images of it appear, through an effect known as gravitational lensing. When the quasar emits a flash of light, it can be seen in the mirage images, but with a slight time lag relative to one another because the paths taken by the light don't have the same length. By measuring this time lag, H0 can be estimated without resorting to a distance ladder. HOLiCOW yielded a result of 73.3 km/s/Mpc, which is very close to the SHoES measurement.
Nonetheless, “before changing the standard model of cosmology, which successfully predicts many observations, we need to be sure that this tension isn't simply due to erroneous measurements”, Rigault warns. In fact, astronomers are well aware that the stars they use as standard candles are not ideal objects. For instance, the fact that some of them appear dim doesn't necessarily mean that they are very distant. Cosmic dust could also be to blame, making stars appear farther away than they really are.
In addition, such objects can experience variations due to their age or their metallicity (the abundance of elements heavier than helium present in them). Astrophysicists try to take all these effects into account and correct for them. However, they are not immune to errors or to unknown effects that may have escaped their notice.
New measurements to the rescue
In the next five years, new observations could provide a clearer picture. The Zwicky Transient Facility (ZTF), an astronomical survey of the entire Northern Hemisphere sky at Palomar Observatory in California (US), has compiled a catalogue of 3000 supernovae which could be used to measure H0. “That's ten times more than what's being done today with SHoES. Besides, all these objects were detected with the same instrument, unlike the other catalogues compiled over some twenty years from different observations. That should eliminate numerous sources of possible error,” points out Rigault, who is responsible for ZTF in France.
The next step will be to calibrate the luminosity of supernovae using red giant stars, a new type of standard candle that some astronomers are now using instead of Cepheids. To do this, Rigault's team have made a request for observation time on the James Webb Space Telescope. If accepted, the astronomers will be able to determine the value of H0. And should the result confirm the SHoES finding, this will further tip the balance in favour of new physics, beyond the standard model.
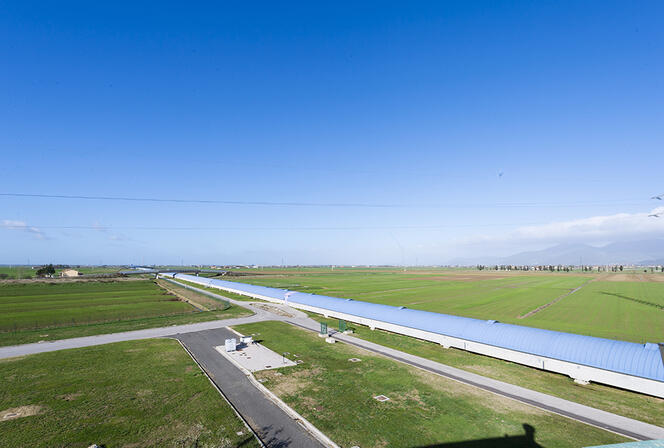
Astronomers also have high expectations from the detection of gravitational waves. In 2017, the observation by LIGO and Virgo of gravitational waves produced by the fusion of two neutron stars, combined with the detection by telescopes of light from the same event, provided a new way of measuring H0, since the distance of the source could be inferred from the waves and its recession velocity from the light. Having recorded just one such phenomenon does not allow for sufficient precision yet, but it is expected that many more will be detected in the near future. The result they will yield should then be key to settling the debate.
Thinking about new scenarios
However, without waiting for the final word, theorists are already thinking about new cosmological scenarios that could explain the Hubble tension. “The models currently favoured are based on the hypothesis that around 380,000 years after the Big Bang, there was an additional ingredient present in the early Universe, which boosted its expansion,” Poulin-Détolle explains. A number of ideas have been mooted, such as the existence of a new type of neutrino, the notion that the laws of gravity were different in the primordial Universe, or the possibility that dark energy played an essential role at that time.
It is this latter idea that Poulin-Détolle is pursuing. He recently proposed a model involving an early dark energy that was greater in the past and contributed to 10% of the expansion of the Universe, before becoming the dark energy whose effects are measured today. According to him, this theoretical solution would have left clear evidence in the first image of the Universe, the cosmic microwave background, which Planck's successors, the Atacama Cosmology Telescope, in Chile, and the South Pole Telescope, in Antarctica, should easily be able to detect in the next few years.
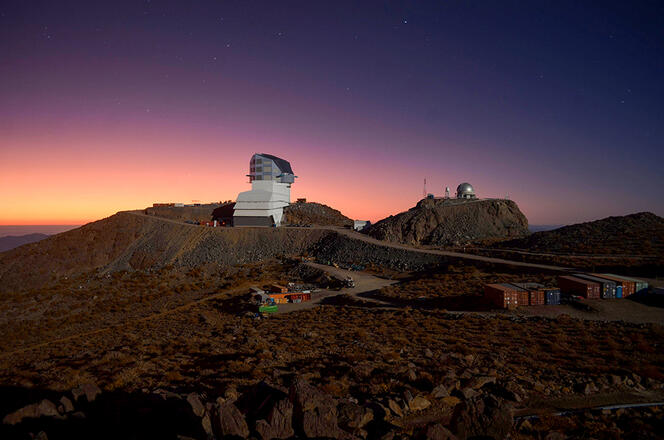
And yet further monitoring, both in space and on the ground, could provide the proponents of new physics with more grist for their mill. The European Euclid space telescope, scheduled to launch in July, and the Vera Rubin Observatory, an American telescope in Chile due to begin observations a year later, will map the sky with unprecedented precision. Together they will catalogue several billion galaxies and provide a detailed picture of the way in which they are structured in the Universe.
For in addition to the Hubble tension, another anomaly is beginning to emerge, albeit not as striking. Observations appear to show that the large galactic structures (galaxies and galaxy clusters) are not as concentrated as the standard model predicts. These major surveys should settle the matter once and for all. And there may be yet more evidence that cosmologists need to rethink their approach. “The breach opened by the Hubble constant is a real opportunity. Although the standard model works very well, we still don't know what its main ingredients (dark energy and dark matter), which make up 95% of the Universe, consist of. Thanks to this crisis, we may be on the verge of solving the mystery of their fundamental nature,” Poulin-Détolle concludes.