You are here
Welcome to the World's Smallest Car Race
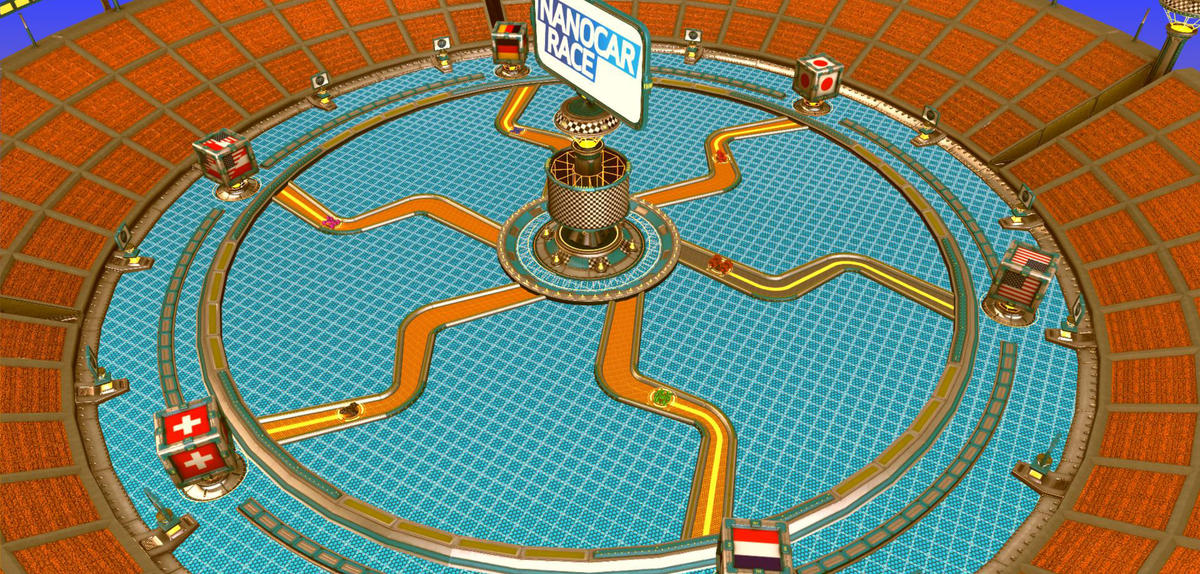
Starting April 28, the entire world will be able to livestream from the CEMES1 for 36 hours, the first nanocar or molecule-car race ever held. But what is a molecule-car?
Christian Joachim:2 It is a molecule equipped with a chassis, wheels and in some cases a visible form of engine. We call it a nanocar because of its size—its wheelbase or the length of its chassis measures between 1 and 3 nm. It is around a billion times smaller than a Formula One racing car.
How do we build these cars?
C.J.: First you have to design them, draw their chemical structure atom by atom. This stage is carried out by theoretical chemists in direct collaboration with synthetic chemists and surface physicists. Then you have to decide where you want to put the wheels, the axles, the chassis and so on... Which means a lot of to-ing and fro-ing between the designer of the molecule, the person who knows how to synthesize it, and the person who will calculate and then simulate on computer the mechanical functioning of this molecule once it has been placed on a surface. Finally, the synthetic chemists get to work.


And to take part in a race, you have to put these molecules on a track.
C.J.: Yes, and there are a few ways of doing this. Practically all molecule-vehicles are synthesized in their complete form, then evaporated and placed on the surface of the track.
However, one of the cars in this race uses a different and very promising method. Instead of synthesizing the entire molecule in solution and placing it on the surface, one team has managed to synthesize only carefully selected chemical groups for the molecule-car in solution and then place them on the supporting surface. They are then able to heat the surface which synthesizes the full molecule directly on location. All competitors will place hundreds of identical molecule-cars near the track in order to change cars if necessary, for example if they have an accident.
So each team has its own track?
C.J.: Yes. Four of the six finalists that will be selected this week will race on the same surface, namely a golden medallion that measures 8 millimeters in diameter, entirely enclosed in an ultra-high vacuum (10-10 mbar) at ultra-cold temperatures (~ 4 K). This gold surface presents several advantages: it is very stable (with little pollution), it has natural folds, or chevrons, on its surface. The chevrons are not very high (0.03 nm), but they form the ‘edges’ of the tracks.
In concrete terms, what size are these tracks and what do they consist of?
C.J.: They must be 100 nanometers in length and contain three straight lines and two turns. The tracks are normally identical—indeed the teams will choose their tracks on their own quarter of the medallion out of thousands of other tracks on the surface. The tracks are between 4 and 6 nanometers wide along the straight lines, but not all of the bends are identical, despite all being at 45 degrees, since they vary in width. We have a track commissioner, Jean-Pierre Launay, who will certify the choice of track and ensure that the total length for each team is indeed 100 nm. In any case, the key thing is to get around the bends, and this is complicated. During the training phase, I spent 5 hours with one team stuck in a turn in the middle of the night.
How do you make the cars move?
C.J.: To make them move—AND observe them—we use the tip of a scanning tunneling microscope (STM). The STM provides images of a surface with atomic precision and can remain focused for minutes on an individual atom. To get an idea of the order of precision and scale, imagine turning the Eiffel tower upside down and using the tip of its antenna to push a small grain of salt. The STM uses a tip that scans a surface. It stabilizes this tip above the surface and measures the intensity of peak electric current. The electronic mechanism controlling the height of the tip adjusts this height along the surface in order to ensure a constant current across each scanline.
Such microscopes are used, for example, to measure the electrical resistance of a single atom or other single molecules. We did this in 1995. It was also used to write with individual atoms—just like Don Eigler did in 1989 with his famous IBM logo. But we can also use it to push a single molecule along a surface as we demonstrated in 1996, or, more recently, to build aan atomic wire atom by atom on the surface of a semiconductor. But we decided to go one step further.
Yes, you can now move the molecule without pushing it mechanically. How is this done?
C.J.: We can now deliver a small amount of energy (a few meV) to one part of the molecule, which can turn a molecule-wheel, or trigger a change in the molecule’s configuration. The tip must thus be placed at the correct position on the molecule to within several picometres, then we apply a current for several seconds at tip-surface strengths around one volt and we can observe the molecule move, after which we stop it and generate an image. The most attentive and experienced competitors can tell whether the molecule has moved from the temporal variations in the tunnel current they are measuring. During the competition, some will even bypass the image generation phase (3 to 5 minutes), but at their own risk, since their car may quite easily have made 10 movements instead of one, thereby straying very far from the track.
The race was created and takes place at the CEMES since we are the only laboratory in the world with a 4-tip STM that has been modified to enable four separate researchers to work on a single surface at the same time, and which thus provides the ‘arena’ for our finalists.
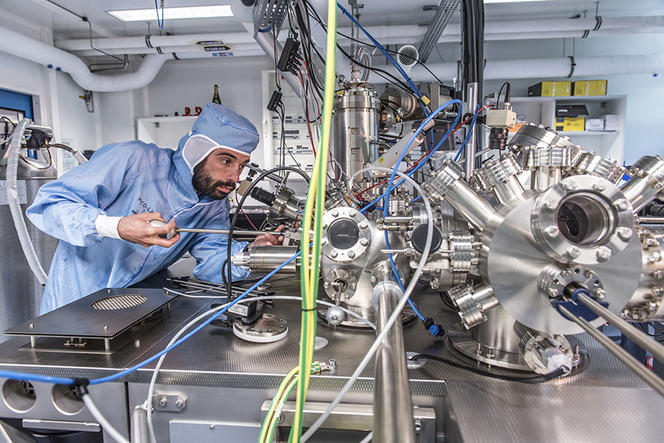
Now onto the competitors. The open call was launched in 2013, and out of 9 candidates, 6 were selected in May 2016. This week, 4 finalists will be chosen to face one antoher on the 4-tip microscope at the CEMES.
C.J.: Yes. These six competitors are the French team from CEMES-CNRS Université Paul Sabatier (France), the team from the universities of Rice and Graz (US and Austria), the Dresden Technical University team (Germany), the MANA-NIMS team (Japan), the Basel University team (Switzerland), and the Ohio University team (US).3 Each team has a ‘pilot’ who controls the microscope tip, and a co-pilot, who manages the overall race strategy. Most vehicles have wheels but some may be a bit different. The Japanese car, for example, has two lateral pedals. All of the competitors have come to train once or twice in Toulouse. The molecule-cars do not all move forward at the same speed but travel on average around 0.3-0.6 nanometers for each pulse of energy. However, their speed per hour highly depends on the number of images they decide to generate.
And speaking of strategy, a number of rules had to be ‘invented.’
C.J.: Not really invented... We based our rules on those of the first automobile race in history: the Paris-Rouen race of 1894. At that time, there were 4 or 5 possible types of engines: electric, mechanical, pedal, etc. The organizers decided to allow all models to enter the race, much like for ours. One of the rules that was added was that if a car breaks down or no longer moves, the competitors must start up one of their spare molecules positioned in advance on the side of the track. This becomes somewhat complicated since the team may decide to deliberately break their car at a convenient point if they see that it is malfunctioning.
It was in fact "the sons of Peugeot frères" who carried off the first prize in this race in 1894 and it is the PSA group that is sponsoring the French team’s molecular car today.
C.J.: Yes, we have had many sponsors for this race. Among the competitors, PSA sponsors the French team, Toyota sponsors the Japanese team and Volkswagen sponsors the German team. Each team was responsible for finding its own race backers.
This helps ensure even more media coverage. After all, a very real objective of this race is to let us discover or rediscover a scientific discipline that is currently booming.
C. J.: Yes, indeed, everyone can readily identify with a car race. This allows many people to quickly and clearly visualize what a molecule is. Through the race, a whole swathe of basic science and of progress in measuring instruments such as the scanning tunneling microscope, is made accessible to the general public. But at the same time, the goal is to show that this story, which began in the field of atomic physics—with the IBM logo for example—now also fully incorporates chemistry and molecule-machine design. Clearly, the idea is also to demonstrate the phenomenal advances that have been made in atomic technology, where real changes will be introduced at pace thanks to our efforts and those of others. For the moment, we have no option but to operate on a flat surface in an ultra-high vacuum using a sort of cable, namely the tip of the STM. But what of the future? We know that we can already increase the surface temperature slightly for some types of molecules without causing them to diffuse. And if we are able today to focus on a single molecule for hours on end (in this case, over the 36 hours of the race) on a work bench consisting of an ultraclean surface, why not elsewhere? In a gel, perhaps, or a liquid? Or possibly on the surface of a cell membrane? Other questions have arisen: how can the functioning of a molecule machine be controlled remotely since an STM tip is somewhat inconvenient. Would it be possible to place a kind of antenna on a molecule which could then act as a receiver for a few photons? The story continues.
Watch the race online starting on April 28: http://nanocar-race.cnrs.fr/indexEnglish.php or on the Youtube channel of the CNRS
- 1. Centre d'élaboration de matériaux et d'études structurales (CEMES)
- 2. Groupe NanoSciences (GNS), CEMES.
- 3. NanoMobile club CEMES-CNRS (France), Rice (USA) & Graz (Austria) Universities, Nano-windmill Company Dresden Technical University (Germany), MANA-NIMS Nano-Vehicle (Japan), Ohio Bobcat nanowagon team Ohio University (USA), Swiss-nano Dragster University of Basel (CH).