You are here
SPIRAL2, the atom factory
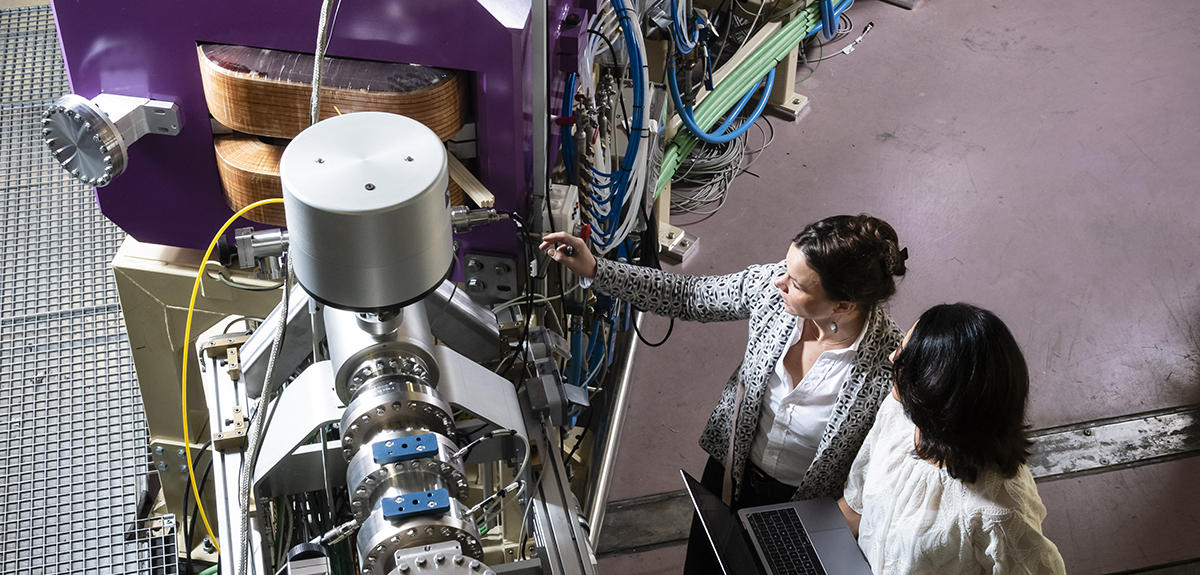
“This is rabbit paradise,” laughs Navin Alahari, director of France's National Large Heavy Ion Accelerator (GANIL), as we stroll around the vast 32-hectare site that hosts the laboratory facilities, on the outskirts of Caen (Normandy). Above ground, there is no hint that, buried 10 metres beneath our feet, one of the world's most powerful particle accelerators will soon be fully operational. Launched in 2005 and officially opened in 2016, GANIL's SPIRAL21 facility and its linear particle accelerator, or LINAC, will provide the opportunity to carry out research like nowhere else in the world.
Right from design to assembly, the project involved the collaboration of a host of nuclear physics laboratories both in France and across the world. Capable of producing beams of light ions (protons, deuterons, helium, etc) as well as heavy ions, with an intensity 10 to 100 times higher than that currently available at GANIL, SPIRAL2 opens up a new era in research on atomic nuclei, both fundamental and applied (in fields such as nuclear medicine, energy and aerospace). “A facility like this one only launches once every twenty years,” says an upbeat Alahari. The goal is to probe the innermost core of matter at the tiniest scales with unprecedented precision.
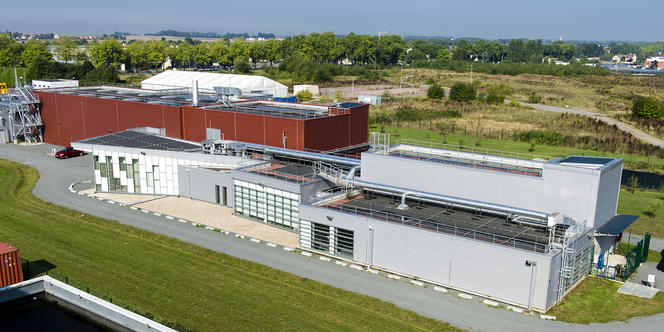
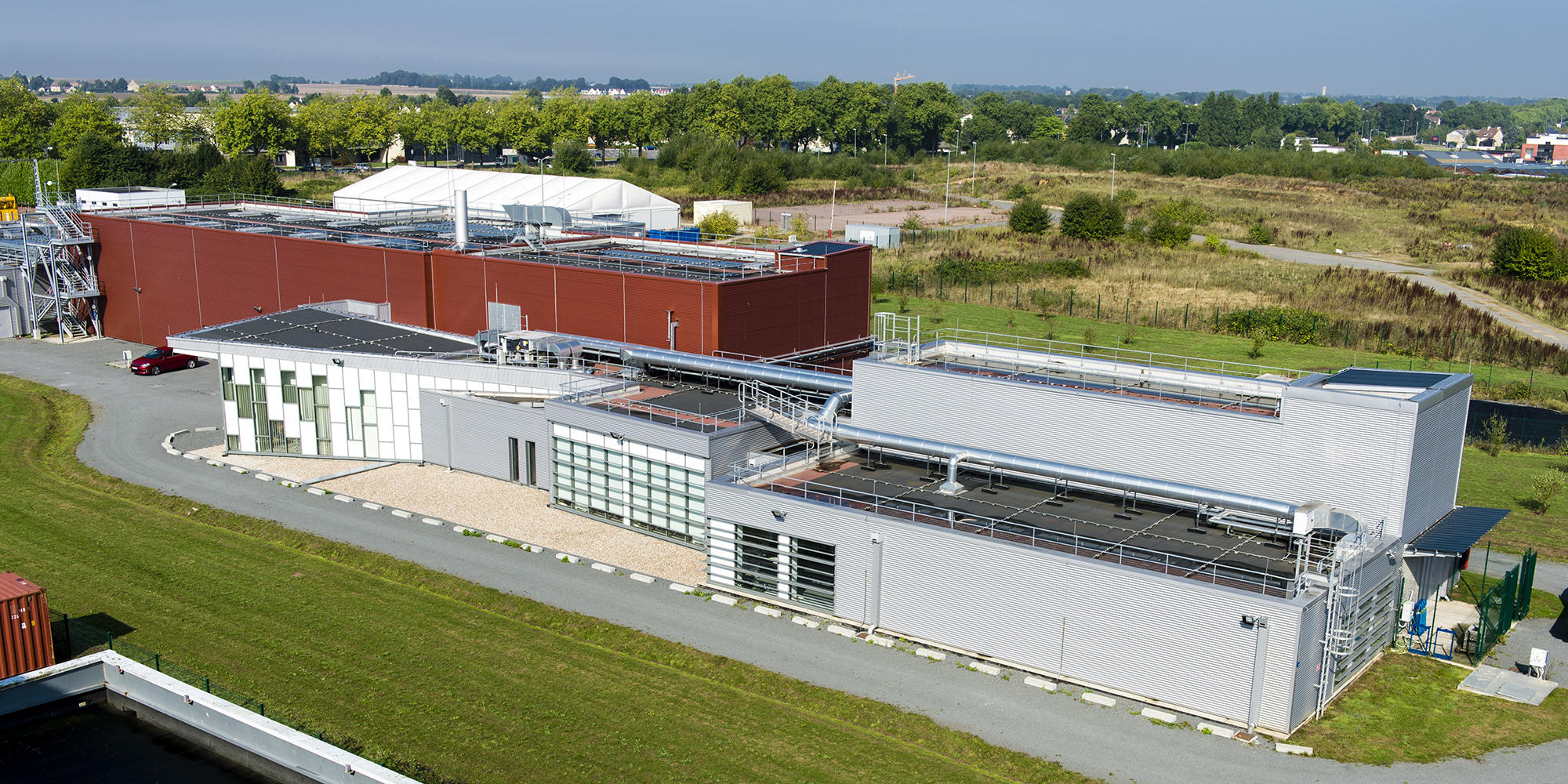
The only accelerator of its kind in the world
Jointly set up in 1976 by the French Alternative Energies and Atomic Energy Commission (CEA) and the CNRS through its National Institute of Nuclear and Particle Physics (IN2P3), GANIL has undergone an uninterrupted process of development. Over the past forty years, physicists from all over the world have come here to smash accelerated ions in order to produce new types of atom. Thanks to this continuous growth, in which SPIRAL2 is a major milestone, it has become one of the world's leading laboratories in the field of ion beam research.
Here, scientists can investigate the structure of atomic nuclei, as well as the reactions (fission, transfer, fusion, etc) and nuclear forces involved. Above all, they can go in search of so-called super-heavy nuclei, which exceed the stability limits of known nuclei, as well as the exotic nuclei produced inside stars. The latter are transitory, unstable and radioactive and don’t exist on our planet (there are 291 naturally occurring nuclei on Earth, while 2 800 have been synthesised in the laboratory to date). At GANIL, over a hundred have already been discovered, synthesised and studied. Now that it is operational, SPIRAL2 will enable scientists to go even further. However, starting up a facility like this is a long process: for months, the new accelerator was methodically tested, component by component, in order to make all the necessary adjustments.
Today, 13 October, the machine has been turned off. The scientists and technicians are carefully preparing the next experiment in one of SPIRAL2's three new halls, Neutrons for Science (NFS). This gives us the opportunity to stroll through the sprawling complex with Bertrand Jacquot, a physicist at GANIL. He knows every inch of these huge buildings, which were custom-built to house SPIRAL2. Equipped with dosimeters, we take a quick look at the original facilities (GANIL's five cyclotrons), before entering the hall that houses the LINAC.
The accelerator peeks out from beneath a tangle of cables, electronics, sensors and superconducting electromagnets. It is here, in a continuous hum of machinery, that, over a distance of some 40 metres, the particles will be accelerated to 75 000 km per second (a quarter of the speed of light) and will bombard tiny targets in the hope of producing the element whose atomic structure the scientists are trying to understand. What happens next inside the machine is like a race against time. The ions are subjected to a series of electromagnetic fields so as to get them to form a beam, accelerate them, and guide them to the adjacent experimental halls. While this operation is already complex enough, the scientists were faced with a number of additional challenges, all centred around one main objective: ensuring both the technical and human safety of the facility.
LINAC's key element, the radio-frequency quadrupole (RFQ)
When the particles exit the ion source (a process invented and developed in Grenoble, in southeastern France) located in a hall upstream, they reach the very first stage: the radiofrequency quadrupole (RFQ), a four-pole electromagnet. Inside this component, made of pure copper and consisting of five sections, each 1 metre long and weighing 1.6 tonnes, a radiofrequency wave bundles the particles into packets – at a rate of 88 million packets per second – so that they can then be injected into the superconducting linear accelerator. Thanks to its complex geometry, the RFQ pre-accelerates all the particles to 4% of the speed of light, slowing down those that are racing ahead and speeding up those that are lagging behind.
This ensures that when they exit the instrument, they are all located in the right place and sent off at the right time to complete the rest of their extraordinary journey. This is a remarkable feat of engineering, as Jacquot explains: “Although every component of SPIRAL2 is a prototype, the RFQ is the key element. It is based on a relatively old idea dating back to the 1960s. But at the time, we didn't have the technological capability to develop it.”
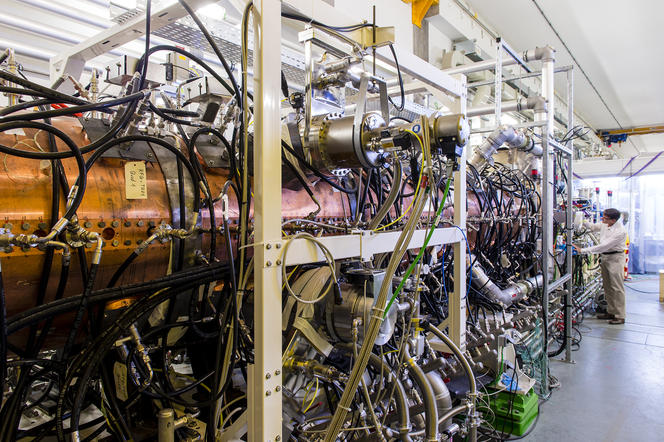
The events that the scientists are trying to observe here are extremely rare. The precision required is so great that getting these collisions to occur has been compared to launching two needles from either side of the Atlantic and hoping they'll collide halfway across the ocean. The greater the intensity of the beam, the higher the probability of observing collisions, simply because these events are much more numerous. To achieve these objectives, the LINAC's beam is a hundred times more intense than that of the original facilities: as many as 3x1016 (30 million billion) particles hurtle through the linear accelerator every second. However, because these particles are electrically charged, they repel one another, which means that some get lost along the way. More efficient than its predecessor thanks to the RFQ, and with greater intensity, power, and complexity, the LINAC can accelerate particles with an efficiency approaching 100% (as compared to 20% for the original cyclotrons), before they continue on their way through 26 accelerating cavities (a French innovation used in large accelerators throughout the world) enclosed in 19 cryogenic modules cooled by liquid helium to a temperature of 4 kelvins (-269.15 °C). In this process of creation and transformation of matter, (almost) nothing is wasted.
Monitoring and calibrating
However, there is no room for improvisation when it comes to reproducing the nuclear reactions that take place in the cores of stars. “With a beam of up to 200 kilowatts2 focused on just a few square millimetres, you could pierce a wall in a split second,” Jacquot points out. Although not every experiment calls for such power, protecting the accelerator itself remains a major concern. “In this case, the notion of time is crucial. The slightest damage, or incident, such as a magnet that breaks down or fails, could result in the destruction of all the material in a few milliseconds,” the physicist explains. The consequences would be extremely serious. Uncontrolled beam loss, for example, could lead to intense irradiation of the machine's components, affecting their lifespan. As a result, the accelerator is constantly subject to in-depth electronic and human monitoring.
No fewer than 120 sensors all along the instrument measure the beam's intensity, energy, speed, position and direction in the tube, which is kept under very high vacuum. Their sensitivity also enables them to detect beam loss (in which escaping particles produce neutrons) by measuring the number of particles at both ends of the beamline. If there is a difference, the machine is stopped. Working 24/7, three operators take it in turn to monitor over 1 000 parameters. “These checks enable us not only to very rapidly identify the slightest potential failure as soon as the beam doesn't behave as expected, such as an anomalous voltage or temperature in an accelerating cavity, but also to fine-tune the device's components,” explains Sébastien Leloir, an electronics engineer at GANIL.
One of the (many) challenges is to adjust the 19 cryomodules that follow on from the RFQ. These contain the 26 superconducting cavities. Between each cryomodule, an ingenious system – the Beam position monitor – is used to observe the beam from all angles and calibrate it very precisely. “This method was partly developed at GANIL,” the engineer points out. “Such a high level of precision is necessary not only to fine-tune the machine, but also for the physics and the interpretation of the results. For instance, when a new nucleus or reaction is discovered, it is essential to know how many beams were directed at the target, the success rate, as well as the intensity and energy that were required. This is because different phenomena may occur, depending on how much energy is used.”
SPIRAL2's 8 000 cables have a combined length of 240 km. They connect the facilities to the building's control centre (known as the Machine Protection System – MPS) which is located at the surface, together with the whole power supply. Since neutrons are extremely damaging to electronics, the MPS had to be moved out of harm's way. There, the source of a potential alarm is identified and safety thresholds are determined, both thermal (if one of the device's components overheats) and radiological (in the event of excess radioactivity). “The electronics are specific to SPIRAL2. There isn't much room for further optimisation with current technologies,” Leloir says.
Producing the heaviest nuclei
The beams of particles, fired at full power and in complete safety, complete their journey in one of SPIRAL2's experimental halls, depending on their characteristics: DESIR (dedicated to exotic low-energy nuclei), S3 (Super Separator Spectrometer), which is still being installed, and NFS (Neutrons for Science), the first one in which scientists are actually working. Today, technicians are busy positioning the detectors for the experiments due to take place the following week in the NFS hall. Jean-Claude Foy, a specialist in instrumentation and experimental techniques, and winner of the CNRS 2021 Crystal Medal, is in charge of operations. Here, they mainly use neutrons, which, as their name suggests, are neutral particles that carry no charge. Electromagnetic fields therefore have no effect on them.
In this long experimental hall, the time the neutrons take to travel from their point of production to the detector, and thus their energy, is measured. “Two people competing in a race will cover a distance of 3 metres in roughly the same time, even if one is running faster than the other. But over a longer distance – in our case, 40 metres – the gap between the two will be much wider, enabling us to measure reactions far more accurately,” explains Héloïse Goutte, GANIL deputy director.
Our visit ends in the S3 hall, still under construction, where scientists will attempt to identify and accurately determine the properties of super-heavy elements, in other words, those that are heavier than uranium. The first experiments there probably won't take place until 2023. “One of the most interesting nuclei is the tin-100 nucleus, which has 50 protons and 50 neutrons. This is the last so-called ‘doubly magic’ nucleus remaining to be studied, and there's a global race on to be the first to do it,” explains Hervé Savajols, S3's scientific coordinator. It was with the aim of winning that race that GANIL acquired the capacity to produce extremely intense beams, as well as a next-generation spectrometer.
For the deputy director of the facility, the key achievement of this extraordinary project has been to unite individual minds and skills around a single goal: that of building extremely complex – and operational – equipment. SPIRAL2 is above all the result of the collective ambition of a vast scientific collaboration, and a highly successful human endeavour.
- 1. Système de Production d'Ions Radioactifs Accélérés en Ligne de 2e génération (2nd-generation production system of online accelerated radioactive ions).
- 2. To get an idea of the orders of magnitude of the power output, 1 kW corresponds approximately to that of an electric iron, and 10 W to that of an electric light bulb. 1 kW = 1000 W. 1 MW = 1000 kW. A power of 1 watt is equivalent to an energy flow of 1 joule per second.
Author
Anne-Sophie Boutaud studies scientific journalism at the Université Paris-Diderot. She holds a degree in history and political science.