You are here
Ceramics Everywhere
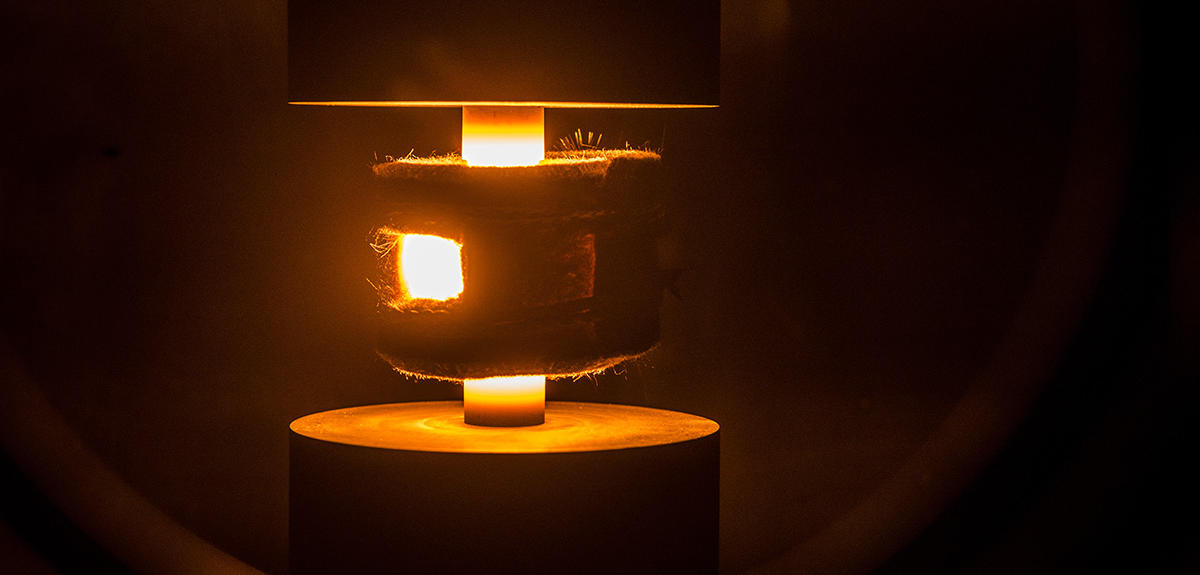
From crockery to building materials and high-tech implants, the ceramics that have been known to us since prehistory now serve as valuable materials in a wide range of fields including aeronautics, medicine, energy, and the environment. The basic process, known as sintering, remains the same: heating a heap of powder at high temperature until its grains agglomerate and form a compact and more or less porous structure. The remarkable properties of this microstructure include porosity, hardnessFermerResistance that opposes the deformation of a material's surface. , thermal resistance, chemical inertness (which is to say environmental stability), and even lightness and transparency. But there is room for improvement! Researchers are focusing on the chemical composition and increasingly precise control of the microstructure of these materials, which have been revolutionary since the Stone Age...
Resistance to extreme conditions...
Like diamonds, ceramics are very hard but have low tenacityFermerResistance to impacts and crack propagation.. In other words, they are not very resistant to impacts, and break easily. To remedy this, materials made up of a ceramic matrix reinforced by ceramic fibers have been developed. These ceramic matrix composites (CMC) combine the solidity and lightness of a composite with the high-temperature resistance of ceramics. The LCTS1 in Pessac (near Bordeaux) has specialized in this area, designing materials, chemical compositions, and varied architectures adapted to the most extreme conditions, such as temperatures exceeding 1,000°C, thermal shocks, oxidizing, and corrosive environments.
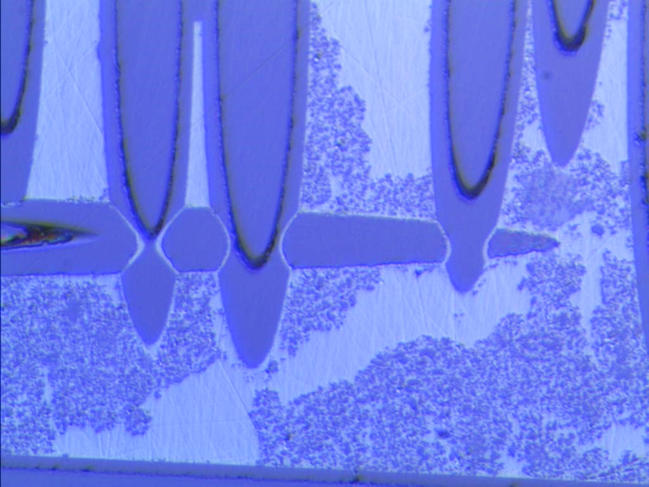
"CMCs have yielded a breakthrough industrial application by replacing metallic parts in aircraft engines. They are two to three times lighter and more resistant, and thus improve motor output while reducing energy consumption and pollution," points out LCTS director Gérard-Louis Vignoles. While these materials are increasingly present in industry, researchers are setting their sights further. They are exposing ceramics to intense and varied conditions (mechanical, thermal, chemical, and tribological stress, both individual and combined) in an effort to develop the next generation of CMCs that can resist even higher temperatures (from 1,500 to 3,000 °C). This type of research, which is also being conducted in the framework of collaborative programs between the CNRS and Onera,2 will be capable of responding to very specific needs, such as the atmospheric reentry of space objects. "However, these are new materials, and we do not have as much feedback as for metals or alloys," Vignoles adds. We must therefore elucidate the fundamental mechanisms that could lead to lower performance and deterioration. The challenge is to maintain resistance to extreme conditions over very long periods."
...almost unbreakable!
Changes must be made to the microstructure of ceramics in order to increase their tenacity. Researchers from the Ceramic Synthesis and Functionalization Laboratory (LSFC)3 in Cavaillon (southeastern France) had the original idea of mimicking the structure of the mother-of-pearl that covers certain shells. "Nacre is made of a fragile material, calcium carbonate, yet the structure it forms is 3,000 times more resistant than the basic bricks of which it is made," explains LSFC researcher Sylvain Deville. This special feature is due to the organization of its components, which resemble a pile of small "bricks" (calcium carbonate) bonded by a "mortar" consisting of proteins. A crack would consequently have to circumvent each brick in order to spread, which slows its progression. To reproduce this structure, researchers demonstrated great ingenuity by using the process of freezing. Concretely, alumina scales (a common ceramic powder) were suspended in water with a "mortar," and then frozen in a controlled manner, thereby self-assembling into a pile, like the calcium carbonate in nacre. After identifying the composition of the "mortar" that provides the final structure with the best balance between resistance and tenacity, the scientists obtained a nacre-like ceramic with a tenacity up to ten times that of its traditional counterpart! The freezing procedure, which involves no additional cost, is very promising for the production of industrial ceramics. The company Saint-Gobain, which co-manages the laboratory, is conducting studies to this effect.

Greater transparency
Glass for watches, armor-plating for helicopter windows... Ceramics have long been known for their great mechanical resistance, and should now become increasingly present in new applications thanks to a more recent property—that of transparency. For the last twenty years, this characteristic has been obtained by producing dense ceramics without porosity, which limits the diffusion of light and gives these materials (most often isotropicFermerAn isotropic material is characterized by identical properties in all directions) transparency and light emission properties that sometimes equal the optical and photonic qualities of monocrystals. The absence of porosity is achieved through the high-temperature and high-pressure sintering of powders, which are often very fine, and have been physically and chemically prepared in advance.
Today researchers are attempting to make these procedures less complex and costly, for instance by producing fluorinated ceramics. The technique developed by Michel Mortier's teams at the IRCP,4 which involves sintering at moderate temperatures, provides a polycrystalline material that is totally dense and perfectly transparent. The patented process and the substance it generates could help design very high-powered pulsed lasers.
Another novel avenue of exploration is to create transparent polycrystalline materials through the complete crystallization of glass. Researchers from the CNRS Orléans-based Cemhti5 laboratory are working on glass whose distinctive composition makes it possible to obtain complete and massive crystallization. "In addition to yielding a material with no porosity, our approach enables homogeneous doping (the insertion of chemical elements that optimize light emission), and is based on the synthesis of glass, a simple and cheap procedure," explains Cemhti researcher Mathieu Allix. With his team he recently developed transparent ceramics made from YAG (Yttrium Aluminum Grenade), by way of room temperature crystallization of glass of the same composition. This plain and inexpensive technique, which provides a material with the high-performance optical and mechanical properties of traditional YAG materials, could have a significant impact in the jewelry, optical lens, and electroluminescent diode industries.

Temporary bone implants
The ceramic implants currently used in bone surgery are fragile, especially when they replace large volumes of bone or are inserted in areas with high mechanical stress, such as the jaw. Hence the notion of designing "temporary" implants that would serve as no more than a prop for the bone until its complete regeneration, after which it would disappear. "Bone regrowth is slow and limited in volume. The challenge is to develop implants that can stimulate it," explains Éric Champion, a researcher at the IRCER6 in Limoges (central France). "We have three ways of doing this: by optimizing the chemical composition of the ceramic to improve its biocompatibility and resorbability; by using additive productionFermerDesigned using computer-assisted drawing from scanner images taken from the patient. The implant is produced via stereolithography, or the accumulation of layers measuring approximately 100 µm in thickness to create tailored implants with a porosity facilitating the invasion of bone cells; and finally by including biological material in ceramics, such as proteins that stimulate cell growth." IRCER researchers are concentrating in particular on the formation of blood vessels, which are indispensable to the development of bone tissue. For example, they have shown that triangular rather than circular pores enable better guidance of blood vessels in implants. A generation of such implants could be used in the growing number of situations that require bone restoration, whether due to accidental or cancerous lesions, or illnesses related to ageing.
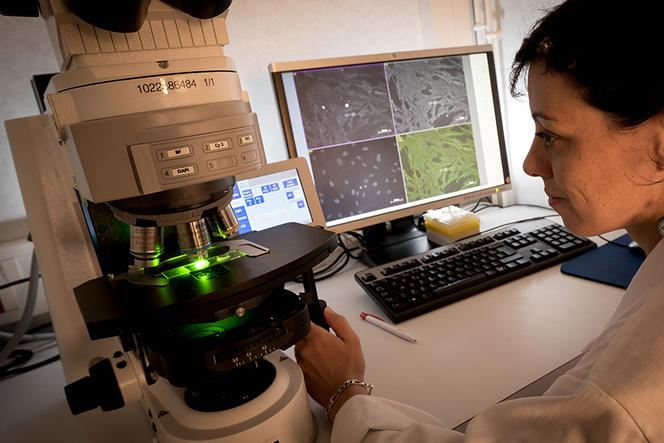
Environment-friendly porosity
Ceramics are porous by nature, and can filter and process liquids and gases. They are most frequently formed of oxides and non-oxides, and are used in many applications, such as the bacterial decontamination of water or the remediation of exhaust fumes.
"Ceramics not only resist harsh usage conditions better and longer than polymer filters, but they also have very fine levels of filtration (from a few nanometers to a few angstromsFermerAn angstrom is equal to 0.1 nanometers, or 10-10 meters (one tenth of one billionth of a meter)), and can serve as a medium for chemical reactions that eliminate undesirable particles or molecules (catalytic functions)," points out Anne Julbe, a researcher at the IEM7 in Montpellier. The development of these meshes requires true materials engineering, and especially the control of both porosity and pore microstructure (size, connections, distribution...), as well as surface properties (composition, electrical charge...). These two parameters determine the filter's selectiveness with respect to its target species, along with the catalytic activity that can be attributed to it.
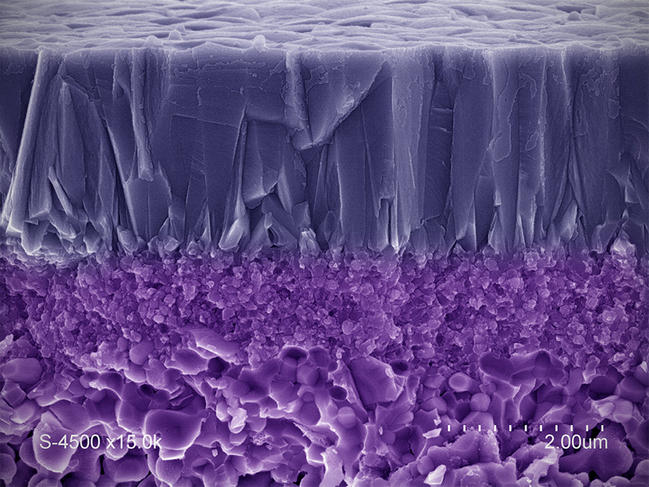
"The challenge is to produce sieves capable of targeting increasingly small species (gas molecules of a few angstroms, for instance), or ones that are present in very low concentration (micropollutants such as endocrine disruptors). This requires developing techniques for analyzing their performance on such scales," adds IEM researcher André Ayral. The laboratory is notably working on gas separation membranes—for instance to isolate hydrogen, the most common raw material found in fuel cells—as well as catalytic membranes that combine filtration and the degradation of harmful species through oxidation reactions (photocatalysis or catalytic ozonation). Such filters, which were created using economical and sustainable processes, will ensure greater control over industrial and urban waste (such as treated wastewater) in the natural environment.
Future nuclear reactors
Uranium oxide pellets, the fuel used in nuclear reactors, are actually ceramics. They provide a material that is resistant to extreme environmental conditions , including temperature (exceeding 300°C), pressure (150 times atmospheric pressure), and irradiation. Preparing the fourth generation of reactors (especially fast-neutron reactors) and addressing the issues they present, such as improved waste security and partial reuse, require advances in these combustible materials.
Researchers are already anticipating their new properties (introduction of new chemical elements, resistance to higher temperatures and neutron flows...). At the Marcoule Institute for Separation Chemistry (ICSM),8 they are seeking to optimally control the microstructure of fuel using a novel observation procedure, based on an electron microscopy technique9 to monitor the formation of the microstructure in real time.
"This approach enables us to experimentally grasp the fundamental phenomena that govern sintering. The data obtained helps improve models for the dynamic simulation of the microstructure by making them more accurate," points out ICSM researcher Nicolas Clavier. "In the end, they are able to ever more closely predict the microstructure of fuel and its evolution." These models are indispensable for anticipating the behavior of future fuels.
Research is also being conducted at the other end of the fuel cycle to develop ceramics that are adapted to the containment of certain types of waste. Inspired by minerals that are naturally resistant to radioactivity, these materials could address the challenges of certain forms of reprocessing, such as very long-term landfills (Cigéo project).
- 1. Laboratoire des composites thermostructuraux (CNRS/Université de Bordeaux/Safran/CEA).
- 2. Office National d’Etudes et Recherches Aérospatiales. The French national aerospace research center. https://www.onera.fr/en(link is external)
- 3. CNRS/Saint-Gobain.
- 4. Institut de recherche de chimie Paris (CNRS/Chimie ParisTech).
- 5. Conditions extrêmes et matériaux : haute température et irradiation.
- 6. Institut de recherche sur les céramiques (CNRS/Université de Limoges).
- 7. Institut européen des membranes (CNRS/ENSCM/Université de Montpellier).
- 8. CNRS/CEA/Université de Montpellier/ENSCM.
- 9. High-temperature scanning electron microscopy technique in environmental mode.
Author
Stéphanie Belaud has been a scientific writer for more than ten years. She specializes in biology, medicine and the environment.