You are here
Sensors, the other quantum revolution
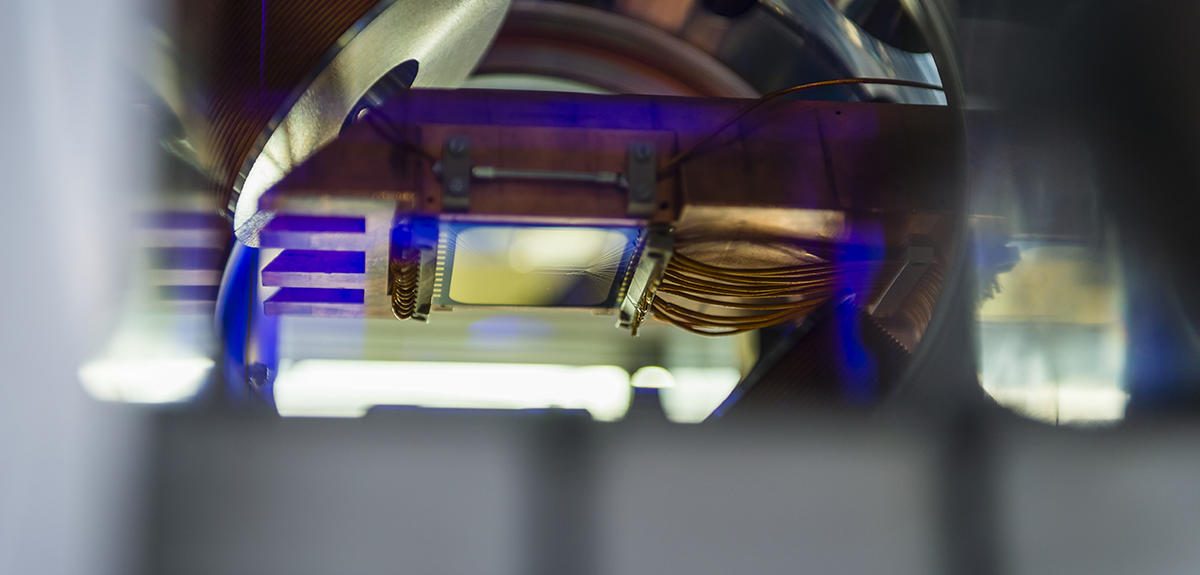
Identifying every pipe and cavity beneath our cities, anticipating a volcanic eruption, or observing brain activity in the most minute detail, these are only some of the tantalising promises made by a new type of instrument with unprecedented sensitivity: quantum sensors. Of all the quantum technologies under development, they are among the most advanced, with some even emerging from the laboratory and reaching the market!
Exceptional sensitivity
As their name suggests, quantum sensors use the properties of quantum physics, the theory that describes phenomena on the atomic scale. Central to these devices are microscopic objects (photons, atoms, electrons, etc.) that physicists can now manipulate at will, and even place within a particular quantum state, which is itself extremely sensitive to the least disturbance in the environment. Quantum sensors are built on the very same principle, which explains their remarkable receptivity to tiny signals of various kinds, such as the gravitational attraction of an object beneath our feet, or the magnetic fields emitted by our brain.
Atom interferometers are the first quantum sensors to have harnessed this potential. These devices, which were initially developed for basic research and metrology, use a laser to cool an atom cloud consisting of a few million particles to very low temperatures, approximately one millionth of a degree above absolute zero. "In these conditions, atoms move so slowly that we can precisely estimate the forces they are subject to, such as acceleration or rotation," explains Arnaud Landragin, who directs the SYRTE laboratory.1
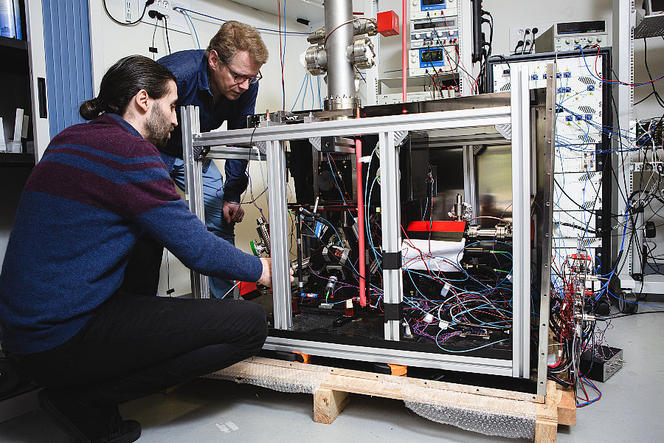
These instruments for example can measure the acceleration of gravity, in which case they are referred to as atomic gravimeters. This is done by allowing atoms to free-fall under the effect of terrestrial attraction. During this process, the particles are subject to a series of laser pulses that place each of them within a quantum superposition – between a state in which it has not absorbed a laser photon, and another in which it has gained speed by absorbing such a photon – before making these two situations interfere. Observations are then made of the interference signal that reflects differences in path between the two states as a result of the acceleration of gravity (g), which makes it possible to work out the latter's value. "The measurement is extremely accurate, as we can detect fluctuations in g on the order of one per billion, or the variation in gravity when rising three millimetres above the Earth's surface!" observes Philippe Bouyer, director of the Photonics, Numerical and Nanosciences Laboratory (LP2N).2
Ultra-stable atomic gravimeters
Such precision opens the way for numerous applications, as accurate measurement of gravity provides invaluable information regarding the composition of the soil. For example, a mass of granite, an oil slick, or an underground water reservoir have distinct densities, and thus contribute to slightly different values for g on the surface. This can facilitate the exploration and management of natural resources. Similarly, by placing such a gravimeter on the slope of a volcano, geophysicists can have a better grasp of its activity, for g will be affected when pockets of magma appear or disappear near the surface. The same is true for monitoring the movement of tectonic plates in zones of seismic activity.
Researchers quickly understood the full potential of this system, and are working tirelessly to extend it beyond the laboratory. This is precisely what Landragin and Bouyer are doing: after developing an optical technique that simplified the functioning of their gravimeter, they decided in 2011 to create the Muquans company, the only one in the world to commercialise such sensors. The French firm has sold a dozen units to date, primarily to geophysical research institutes. One of its measuring instruments was even installed on top of Mount Etna this summer as part of the Newton-g European project, in order to monitor magma movement with the ultimate goal of connecting it to the volcano's activity, and one day anticipating its eruptions.
Increasingly compact devices
The Muquans instrument, which consists of a cylinder measuring 70 cm in height, and a second and somewhat larger module for electronics and lasers, is relatively compact. In terms of size, it is bettered by only one other type of gravimeter, which is made up of a mass hanging at the end of a spring. Easily transportable, the latter is now the most widely used in the field. "The problem is that its measurements drift over time, so that it has to be calibrated regularly. The atomic device, on the other hand, provides an absolute measurement that remains stable, since it is based on the laws of quantum physics," explains Landragin.
As regards precision, another type of gravimeter, also absolute – consisting of an optical interferometer one of whose mirrors enters free fall – offers the same performance as its atomic equivalent. "The downside is that its mechanical parts are subject to considerable wear and tear, unlike the atom gravimeter, which requires little maintenance. The latter can therefore be used to make continuous readings over long durations." This is a major asset for monitoring natural phenomena in the long term.
These advantages explain why geophysicists are increasingly adopting this new system, especially as its size will shrink in the future. "In the next four years, our instrument's weight will be halved to 50 kilos instead of today's 100. This will further expand its applications," enthuses Bruno Desruelle, president of Muquans.
Gradiometers for detecting buried cavities
And there is more. Laboratories and companies such as Muquans are working on an improved version of the gravimeter, known as a gradiometer, which is not affected by tremors in the ground that can disrupt measurement. This can be achieved by using two atom clouds exposed to the same vibrations, but falling from two different heights. Comparing the signals from the two clouds makes it possible to eliminate noise interference, all while obtaining a measurement of the gravity gradient. "The gradiometer can detect small masses located at shallow depth, whereas the gravimeter is sensitive to large masses at great depth," Desruelle points out.
This sensor could make life easier for civil engineering and construction companies, which must conduct lengthy and costly field studies to identify a former mine or other dangerous underground structure, and often have to dig to locate pipe networks or cables beneath street level. A gradiometer would help them save an enormous amount of time – and money.
Accelerometers that do not drift
With more than one string to their bow, atomic interferometers could also be used to develop a navigation system that would continue to function even in the absence of a GPS signal, a priority for the armed forces in particular. Such an autonomous mechanism already exists – involving accelerometers and gyroscopes to measure, respectively, the acceleration and rotation of a vehicle – in order to continually determine its direction and speed of movement, and thereby work out its position. But like spring gravimeters, these instruments drift over time. For example in an airliner, the instability of these inertial sensors translates into an error of approximately one hundred metres after one hour of flight. "Because they are intrinsically stable, atomic accelerometers and gyroscopes allow for much more accurate guidance," Bouyer stresses.
These atomic inertial measurement units are in use today, but only in laboratories. They are still too big to be placed on-board vehicles, and are not yet resistant enough against vibrations, which are frequent on boats and airplanes.
Several avenues are being explored to improve them. At Thales, the French tech giant that is developing multiple quantum technologies for various fields of application, efforts are underway to miniaturise the device by relying on the innovative technique of atom chips. This once again involves laser-cooled atoms, which instead of being subject to free fall, are magnetically trapped on a chip, where they are manipulated using radio waves produced by electrical microwires. "We hope this will enable us to create an inertial sensor with a volume of just one litre by 2030," enthuses Daniel Dolfi, in charge of the physics group at Thales Research and Technology.
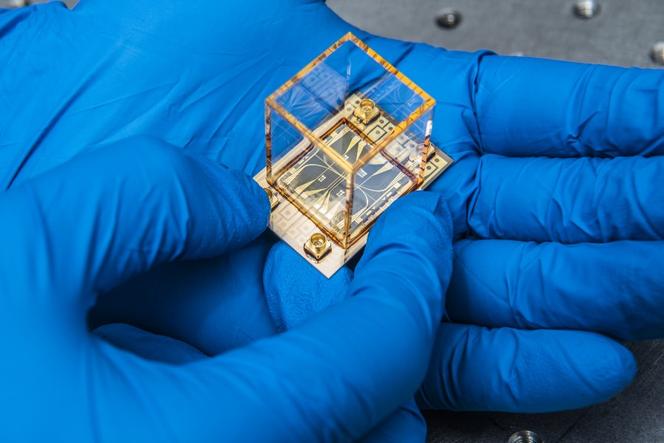
Synthetic diamonds to measure magnetic fields
Another extremely promising quantum sensor is known as an NV (nitrogen vacancy) centre, a microscopic defect lodged in synthetic diamonds that can detect very weak magnetic fields. These impurities, which consist of a nitrogen atom and a vacancy instead of two carbon atoms, behave like single atoms: when excited by a green laser they emit red light whose intensity depends on the spin state – a quantum magnetic moment that can be represented as a small magnetic needle, and is therefore sensitive to a magnetic field – of the electrons trapped in their vicinity. By sending microwaves at the right frequency, one can create quantum superposition states, and thereby record a change in the quantity of light emitted. However, the presence of an external magnetic field will shift the position of spin states, and thus modify what is known as its resonance frequency, by a value that is proportional to the field's intensity. By measuring the amount of red light received, the value of the magnetic field can be worked out precisely.
"The first advantage of NV centres is that they are simple to put in place, as they function at room temperature, and do not require heavy cryogenic equipment, as is the case for other systems. This opens the way for numerous applications," stresses Thierry Debuisschert, in charge of NV centres at Thales. For instance, some existing magnetic field sensors, called Squids, offer greater sensitivity than NV centres, but they must operate at a very low temperature.
Diamond magnetic microscopes
The other advantage of these artificial atoms is their size. Today, it is possible to produce tiny diamonds of a few dozen nanometres that contain a single NV centre. Putting this mineral at the end of a silicon tip and bringing it very close to a material means obtaining another type of microscope, one that can identify the magnetic properties of an object in great detail when swept across its surface. "This microscope can make very fine measurements of a material's magnetic field, with a resolution of a few dozen nanometres. It's quite unique," says Vincent Jacques from the Charles Coulomb laboratory (L2C),3 who with his team, in 2012, produced the first magnetic images using NV centre microscopy.
Since this demonstration, several start-ups have set out to develop these new-generation microscopes, with the first commercial prototypes already available on the market. This is so far the most advanced application for NV centres.
These instruments should enable huge progress in the field of spintronics, a branch of electronics that uses the spin of electrons and not just their electrical charge. A number of materials are being considered to improve components for this electronics of the future. Identifying the best candidates requires perfect knowledge of their magnetic properties. "The NV centre microscope is the ideal tool in this effort, for it alone can probe, at room temperature, nanometric-scale magnetic fields at specific magnetic moments," Jacques points out.
A new imaging procedure at the cellular level
Applications are equally promising in biology and chemistry. Since NV centres are controllable at room temperature and the diamond is not toxic, it is possible to directly place, without causing any damage, cells or molecules on the surface of a stone measuring a few millimetres, in which multiple NV centres have been embedded to boost its sensitivity to magnetic fields. The idea is to calculate the nuclear magnetic resonance (NMR) of these samples, which is to say the oscillating fields produced by the spin of atomic nuclei. "The advantage compared to other NMR techniques is sensitivity to a few spins, and even to one only," points out Thierry Debuisschert, who coordinates the Asteriqs European project, whose goal is to explore every avenue for the use and improvement of NV centres. “This makes it possible to characterise single cells or molecules.”
Research has already begun to this end, and there are hopes that these sensors can one day serve to analyse brain activity in detail, or to closely study the structure of a protein in space (which determines how it functions) – a substantial advantage for designing new drugs.
Improving telecommunications
A highly novel application for these quantum sensors is being developed at Thales. "Rather than rely on NV centres to detect a magnetic field, we could do the opposite: apply a magnetic field to a diamond in order to identify the microwave frequencies present in the environment," Debuisschert adds. The device subsequently becomes a spectrum analyser, which can simultaneously recognise hundreds of different frequencies in the field of radar waves. This instrument could eventually enable the military to intercept communications or identify a threat (the signature of a radar or a missile). In everyday life, it would improve mobile telecommunications, since continual analysis of the frequency bands being used makes it possible to reassign them in real time to a particular operator, as necessary.
The laboratory prototype developed by the French company has already shown the worth of this technique. The team is now working on reducing its size and increasing its sensitivity, especially by making optimal use of the red light emitted by the diamond, and by improving the crystal's quality. The goal is to offer a sensor worthy of the name within the next five years.
These defects in diamonds will continue to generate interest, all the more so as scientists are beginning to explore their capacity – once again using their spin state – to measure tiny electric fields and variations in temperature and pressure. In short, they’re aiming at the ultimate quantum sensor. With its many facets, no doubt this instrument has a bright future.