You are here
Measures: the Great Shift

The universe of metrology is undergoing a true revolution. The “Big K,” or the international prototype kilogram (IPK), the platinum-iridium cylinder conserved at the International Bureau of Weights and Measures (BIPM) in Sèvres—which has defined the unit of mass since 1889–will be retired. The old standard will make way for a new definition of the kilogram, based on the Planck constant from quantum physics. This is, in any case, what the General Conference on Weights and Measures (CGPM) should officially annouce during its 26th meeting, which will take place in Versailles from November 13-16, 2018.
And that is not all, as the venerable institution will take advantage of this occasion to redefine three of the other seven units1 in the International System of Units, the famous SI, once again based on fundamental constants. A definition explicitly founded on these constants will also be adopted for the complete series. All of this will confer a truly enduring and universal character on these base units, as part of a long process to provide a coherence that is unprecedented in the history of metrology.
Units that have become inaccurate
It was clearly time to re-establish order. “All the definitions for units had become more or less pathological,” says quite simply Marc Himbert, of the National Conservatory of Arts and Crafts (CNAM). Let’s be the judge: while science and industry have entered the era of the nanoworld, all of the mass references on the planet are calibrated today based on an artifact whose mass has slowly varied by approximately 50 micrograms in relation to that of its copies. Leaving that aside, “imagine if the mass standard is dropped, that would be the end of the kilogram!” remarks François Nez of the Kastler-Brossel Laboratory (LKB).2 And one by one the units that depend on it, such as the newton, joule, and watt.
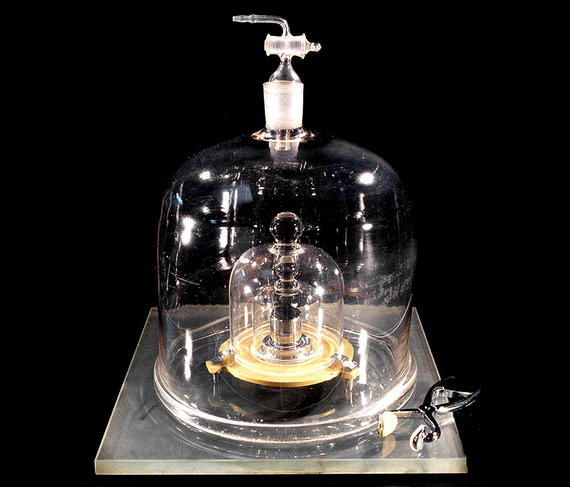
The kelvin, a unit of temperature, is currently defined as a fraction of the thermodynamic temperature of the triple point of water—in which water coexists in equilibrium under the three phases of solid, liquid, and vapor. However, this definition depends on the quality of the water (impurities, isotopic composition) used to implement it. What’s more, as it’s linked to a particular temperature, it is not well adapted to measuring temperatures below 20 kelvins (-253.15 °C) or above 1,300 kelvins (1,026.85 °C).
And what to say of the ampere, the unit for electrical current, which is defined based on the mechanical force between two infinitely long wires, one meter apart, in which a current circulates! For a number of decades, professionals in the field of electricity have in practice traded it for a definition involving quantum processes. As Marc Himbert sums up, “we were facing a four-part problem of coherence, permanence, universality, and precision.” These four criteria are what have dictated the work of the CGPM since it was established in 1875 with the signing of the “Metre Convention,”3 no less than the oldest international treaty in effect today.
An ideal of universality
As Matthias Dörries of the Henri-Poincaré Archives4 at université de Strasbourg explains, “during the nineteenth century, owing to the industrial revolution and the emergence of a vast international market, major nations grew aware of the need to implement a system of common norms.” In a word, this meant ensuring that a kilogram of sugar was the same in Paris, New York, or London. Just like today, the objective was to establish confidence in measures, the only one that could develop, on a reliable basis, scientific knowledge, political decisions, and commercial exchange.
In addition to these practical considerations, the normalization of units of measure was also driven by the previous century’s ideal of universality, personified in particular by the French Revolution. In 1791, the Constituent Assembly decided to define “a unit that bears nothing arbitrary or particular to the situation of any people on the globe.” This proclamation was behind the scientific saga of the two astronomers Delambre and Méchain, who for a period of seven years measured a fraction of the Paris meridian between Dunkirk and Barcelona, from which they deduced the length of a meter, thereby laying the foundation for the metric system, the ancestor of SI. This astounding adventure brought together fundamental science, technology, and politics, which are still connected today.

It is in this spirit that the CGPM duly noted in 2011 the intention of the International Committee for Weights and Measures (CIPM)5 to propose a revision of the SI, and in 2014 adopted a resolution on the future revision. The principle of this revision is to refound the SI on the most universal and intangible of what physics can offer today: fundamental constants, especially those from the physics of the infinitely small known as quantum mechanics, in which the regularity of phenomena and their precision offer unparalleled references. “The driver of the change underway is certainly the emergence of quantum mechanics in the world of metrology,” confirms Christian Bordé, president of the Standing Committee for Science and Metrology at the Académie des sciences.
The consecration of quantum mechanics
Concretely, quantum mechanics made its entry in SI in 1967, with the redefinition of the second in reference to the rate of transition between two levels of the cesium atom. Then in 1983, the meter became the length travelled by light in a fraction of a second, a unit that is subsequently deduced from the realisation of the second and a fixed value for the speed of light. The reform currently underway is in keeping with this perspective.
The kilogram will soon be redefined according to the Planck constant, h (see graphic). It is actually the product of energy by time, and the energy is connected to mass through the equation E = mc2. The kelvin will be redefined according to the Boltzmann constant (k), which is connected to measurement of the thermic agitation of a body’s fundamental components. The ampere, which is simply a charge by unit of time, will be tied to the elementary charge (e). Finally, the mole, a unit of quantity for mass, will be directly defined by fixing the Avogadro constant or number (NA).
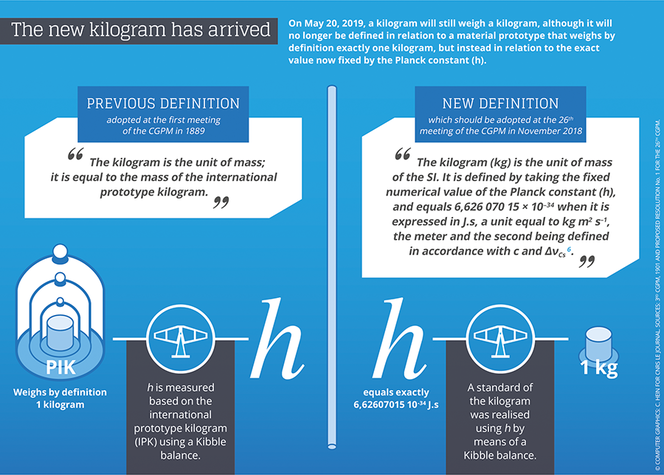

International experts weighed a number of criteria to reach this point. What is the most coherent way of defining the units in view of the knowledge of fundamental physics? Is it possible to physically realize the definitions being considered? How should these realizations then be used to disseminate the units with users? In the case of the ampere, for example, a part of the discussion was to determine whether it should be defined based on the dielectric vacuum permittivity or the elementary charge. “The first possibility was defended by opticians and physicists of diluted environments, the second by physicists of condensed matter,” points out Himbert. “In the end the second group won out, in the sense that it is more consistent with the way electricians effectively measure an electrical current.” This is an illustration of the fact that in matters of metrology, practical questions have at least as much weight as fundamental considerations.
Changing everything… so that nothing changes
If this is indeed the case, should we expect instantaneous upheaval? “Very clearly not,” indicates Himbert. And for a simple reason: everything was done to ensure maximal continuity between the old and the new system. The values of fundamental constants that help define the new SI were fixed once and for all after being measured very precisely—and thus with very low uncertainty—according to the former definitions. The uncertainty of measures henceforth involves the old reference standards.
If that is the case, why change references? Quite simply because once the kelvin is based on a fundamental constant, its definition will no longer involve a particular temperature, concretely avoiding the propagation of errors calibrating thermometers, as one moves away from water’s triple point. The same is true of the kilogram, which will henceforth make no reference to a material standard, etc.
“The primary beneficiaries of the new kelvin should be industries affected by high temperatures,” points out Himbert. And that’s no small matter, as “60% of the sensors used in industry are temperature sensors.” Laboratories in fundamental research working with very low temperatures will also benefit, as will those focusing on the properties of matter on the smallest scales. This will have consequences that are impossible to anticipate. “It would have been almost impossible to develop GPS without the redefinition of the meter in 1983, although this redefinition did not prefigure the development of this technology in any way,” emphasizes Himbert.
A reform to renew confidence
Beyond this, “the new SI will renew our confidence in fundamental units and their universal character,” adds Christian Bordé. It has nevertheless met some scattered resistance. “It took a generation for the metric system to be imposed through school,” reminds Matthias Dörries. “And for some applications, we still count by twelves!” And not just in the marketplace.
On September 23, 1999, the Mars Climate Orbiter probe crashed on Mars. The cause was flight software that expressed the thrust of microthrusters in Anglo-American units of measure, whereas the navigation team’s flight software that was receiving this data in order to calculate trajectory corrections was expressing them in units from the metric system…“While this anecdote is edifying, it does not call the SI into question, but instead the use one makes of it,” Himbert remarks.
In any event, will the new SI, which is based on the most fundamental physics, be the ultimate system of units? Nothing is less certain. For many scientists, the candela, a unit of luminous intensity, does not belong in the system at all. “There is nothing fundamental about the candela,” admits Himbert. “Yet it is very important for the lighting industry, with real economic, security, and health considerations.” The same is true for the mole, which is defined based on the number of atoms contained in a gram of carbon 12, and that according to some has no more meaning than a dozen for eggs, despite being widely used in all types of chemistry and their applications.
Yet that is not all. Some point out that the second remains dependent on the properties of a particular atom, cesium. “On paper, one would have to introduce G into the SI, the fundamental constant of gravitation, in order to define the second. This would be the most natural way to proceed for a physicist,” pleads Bordé. “Except that today there is no way of measuring G with the requisite precision.” Conversely, the definition in effect permits a relative uncertainty of 10-16, which no other unit can claim, subsequently putting the second atop the entire SI edifice. “It makes no sense to have a fine definition that is impossible to realize,” argues Noël Dimarcq from the laboratoire Systèmes de référence temps-espace.6 “The strength of the second’s definition today is its precision.” Without taking into account that the unit of time is currently the only one we can transfer from one point of the globe to another by satellite or fiber optics.
“At best, the SI reflects our knowledge of physics on the fundamental and experimental level at a given point in time,” sums up Nez. “From this point of view, the new system is the best one that we can equip ourselves with today,” concludes Bordé. Which largely justifies doing away with “Big K.”
__________________________________________________________
The metamorphoses of the meter
Just like the kilogram, ampere, kelvin, or mole, the definition of the meter and the second will be changed during the 26th meeting of the CGPM. However, the redefinition of the meter will be more superficial than that of the kilogram, as it will consist of elucidating the physical constant on which the unit of length is built, in this case c, or the speed of light in a vacuum. As a result, the meter has been defined until present as “the length of the distance traveled by light during 1/299,792,458 of a second.” Beginning in 2019, a rigorously equivalent definition will take effect, one known as an explicit constant, in which the meter will be “defined by taking the fixed numerical value of the speed of light in a vacuum, c, which is equal to 299,792,458 when it is expressed in m/s, the second being defined according to ΔνCs.”
Defined starting in 1791 as one ten-millionth of the length of the distance between the Equator and the North Pole, the meter was measured with precision by the astronomers Delambre and Méchain. It was from this definition that the first material standard of the meter was realized. The meter was also the first unit to be redefined based on a constant of atomic physics. Before being based on the speed of light (fixed in 1983), the CGPM abandoned in 1960 any reference to the standard meter constructed in 1889, and defined the meter based on the “wavelength of radiation in a vacuum corresponding to the transition between the levels 2p10 and 5d5 of the krypton 86 atom.”
- 1. The meter, kilogram, second, ampere, kelvin, mole, and candela.
- 2. CNRS/ENS/UPMC/Collège de France.
- 3. Available at the site: www.bipm.org
- 4. CNRS/Univ. de Strasbourg/Univ. de Lorraine.
- 5. Under the authority of the CGPM, the CIPM directs and oversees the activities of the BIPM.
- 6. CNRS/Observatoire de Paris/UPMC/LNE.
Share this article
Author
Born in 1974, Mathieu Grousson is a scientific journalist based in France. He graduated the journalism school ESJ Lille and holds a PhD in physics.
Comments
Log in, join the CNRS News community