You are here
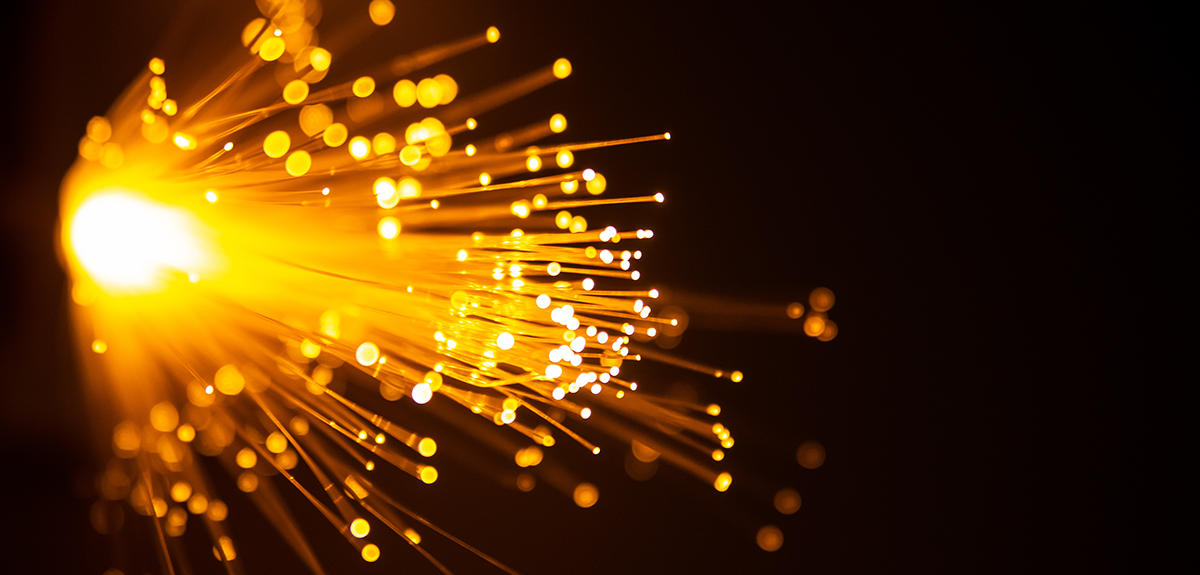
Declared the International Year of Glass1 by the United Nations, 2022 coincides, by sheer coincidence, with the centenary of Brillouin scattering, a significant breakthrough in optics.2 Like Raman scattering,3 which describes the propagation of light through molecular vibrations, this other optical phenomenon pays tribute to Léon Brillouin (1889-1969) of France, the first physicist to reveal it. While the researcher's discovery generated little enthusiasm when it was published in 1922, it was remarkable nonetheless, as pointed out by Jean-Charles Beugnot, a CNRS researcher at the Femto-ST Institute:4 “In his purely theoretical article, which in essence explains that light propagates in all directions thanks to acoustic waves, Brillouin predicted that this scattering could be accompanied by a change in frequency. However, at the time most scientists specialising in optics believed that only fluorescence could create such a shift.” While he was not awarded the Nobel Prize in Physics for his theory, which the Russian Evgenii Gross (1897-1972) demonstrated in 1930, Brillouin foresaw its potential for application, such as the ability to determine the mechanical properties of materials.
A century after it was discovered, Brillouin scattering still fascinates scientists who work on fibre optics. “While specialists in spectroscopy strive to make the most of the phenomenon, researchers in telecommunications and high-power photon lasers want to eliminate it at any cost, as this scattering limits the power transmitted by optical fibres,” summarises Beugnot, who uses the Brillouin interaction to develop new generations of acoustic sensors. “By having light circulate in optical fibres whose diameter was reduced by a factor of 100 to 1 micrometre (µm), or a millionth of a metre, it is possible to generate acoustic waves not just inside the fibre but also around it.” This “non-linear” effect could eventually be used to measure pollution in groundwater.
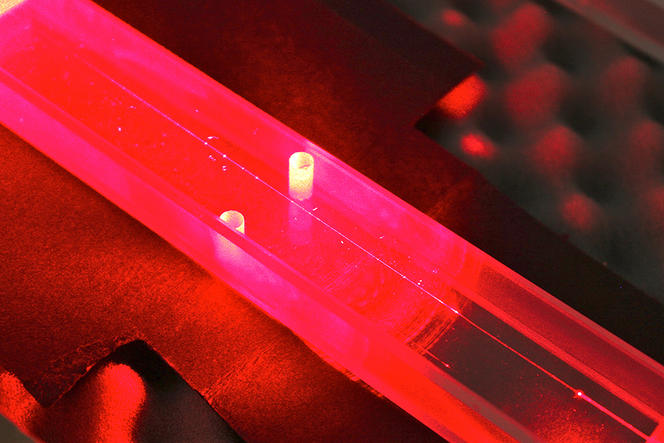
Increasing transport capacity through multiplexing
In most people's minds, optical fibres are generally associated with the transport of large volumes of information over very long distances. This feat was made possible in the early 1970s thanks to the development of processes derived from chemical vapour deposition (CVD). This technique can produce an extremely pure bar of silica known as “preform”. The single-mode optical fibres made from this preform can transmit the same light signal over a hundred kilometres. By contrast, light does not propagate beyond twenty meters in the glass used in our bay windows. There is no comparison between the flow of data that can be handled by an optical fibre and a copper wire. “The maximum capacity of a single-mode optical fibre used in a transoceanic cable can reach approximately 100 terabytes per second (Tb/s), which is equal to the transmission of 500 Blu-ray discs per second,” explains Laurent Bigot, a CNRS research professor at the Laboratory of Physics of Lasers, Atoms, and Molecules (PHLAM).5
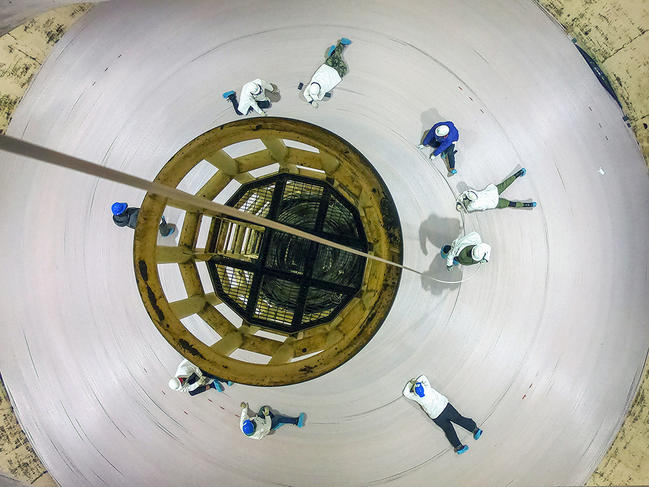

However, with the exponential growth of Internet traffic, the information highways in the form of submarine or terrestrial telecommunications cables could soon be saturated. To limit this risk, over the last decade the scientist and his team have been developing a new generation of multichannel fibres in partnership with the company Draka, a subsidiary of the Italian group Prysmian, a global leader in telecommunications cables.
“By taking advantage of spatial multiplexing, which increases the number of optical paths in the same volume of glass as a single-mode fibre, light transmission can be multiplied by one hundred,” underscores Bigot, who relied on the FiberTech Lille platform, based at the Research Institute on Software and Hardware Devices for Information and Advanced Communication (IRCICA),6 to accelerate the development of these innovative fibres.
Bigot's team is using this very same technological platform, which was intended to bring industrial actors and researchers together, in an effort to identify new applications in the field of photonics. The research being conducted with the start-up Lightcore Technologies, founded in 2019 by engineers and scientists from the Fresnel Institute,7 the XLim Institute8 in Limoges (southwestern France), and the PHLAM, aims for instance to develop new- generation endoscopes: “This involves developing a biomedical imaging technique that is as non-invasive as possible, using hollow-core fibres tipped with a silica bead that serves as a lens,” details Bigot. The early detection of some cancers could soon be a reality by injecting the beam from a pulsed laser into the core of these fibres previously placed in contact with an organ or living cells.
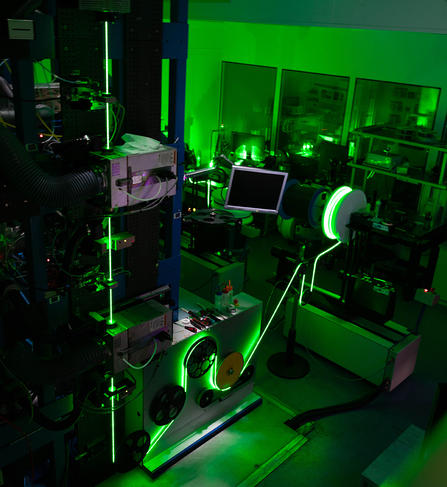
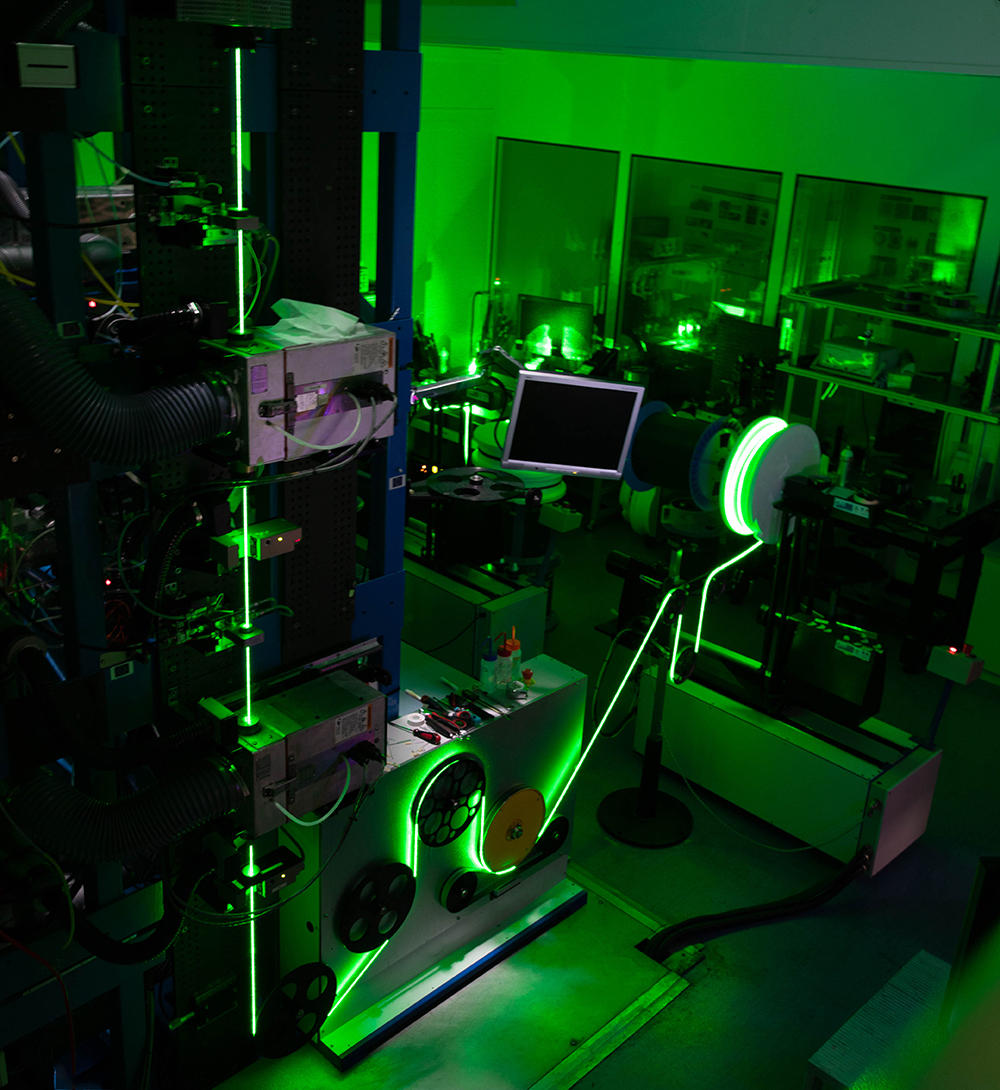
Pooling research to stimulate innovation
This type of collaboration, which brings together a number of laboratories around the same project, is one of the distinctive features of French research on optical fibres, as well as one of its primary advantages. On the national scale, the academic teams involved in the development and production of innovative fibres are part of the GRIFON9 Scientific Interest Group (GIS). “Through the annual meetings it organises, the GRIFON GIS enables our community to keep up to date with ongoing developments and the technological resources available in various research centres. By fostering emulation between laboratories, this GIS also promotes shared research, which is often the first step towards more ambitious collaborative projects," adds Bigot, whose laboratory was behind this Scientific Interest Group, along with the Nice Institute of Physics (INPHYNI)10 and XLim.

Over time, the researchers who develop new optical fibres – crystalline, microstructured, oxide, fluoride, chalcogenide glass fibres, etc. – have also established close ties with national industrial actors in telecommunications and laser systems. “This privileged relationship between academia and the business world helps to better identify upstream research subjects likely to take concrete form as future technological developments with these industrial players,” asserts Wilfried Blanc, a CNRS research professor working at the INPHYNI on a new optical fibre concept containing nanoparticles. This innovation recently demonstrated it could offer new opportunities for developing sensors. “Transparency is a watchword in the development of optical fibres,” Blanc points out. “We are going against the grain by using the light scattering caused by the presence of nanoparticles.”
The nanostructured optical fibres designed by the researcher could measure variations in temperature, stress, and irradiation using ionizing radiation. When attached to a hypodermic needle, such a fibre could, for example, more precisely target the injection zone for an epidural, in order to enhance the effectiveness of the anaesthetic product. Thermal surgery is another promising field of application for nanoparticle-doped optical fibres.
This cutting-edge surgical method raises the temperature of diseased tissue, such as a cancerous tumour, in order to destroy it. “Using this type of optical fibre allows us to precisely map temperature, thereby limiting the risk of overexposing healthy nearby tissue to heat,” Blanc adds.
Counting on infrared to expand applications
The northwestern region of Brittany is a French hub for photonics, with numerous state-of-the-art laboratories and research groups studying the production of next-generation optical fibres. This is true of the “glass and ceramics” team at the Rennes Institute of Chemical Sciences (ISCR),11 which has been developing optical fibres from chalcogenide glass since the 1990s. Because it contains chemical elements from the chalcogen family, such as sulphur, selenium, and tellurium, this type of glass can transmit infrared radiation up to a wavelength of 20 µm. “The production of optical fibres from chalcogenide glass is a delicate process because it requires a preform free of any contamination, which is obtained, among others, from a succession of purification stages,” says Catherine Boussard-Plédel, a CNRS research engineer at the ISCR. Initially focused on mid-infrared evanescent wave spectroscopy, the early work of this specialist in chalcogenide glass led to the creation of the start-up Diafir in 2011.
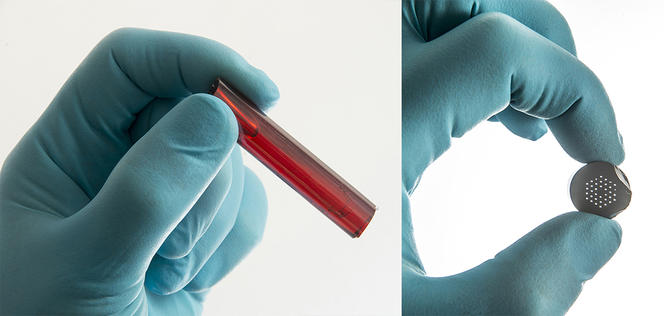
The medical sensors developed by this company are designed to screen – using a simple drop of serum – for individuals suffering from non-alcoholic steatohepatitis, a liver disease whose early diagnosis remains complicated. Applied to the analysis of synovial fluid, the same sensors can already evaluate the risk of osteoarticular infections within minutes.
“These diagnostic tools use evanescent wave spectroscopy to record the infrared signals of a wide range of molecules, doing so in real time and through simple contact with the substance being analysed,” the scientist explains. Far from being limited to the medical field, her research on chalcogenide glass fibres offers many other prospects for application: detecting the bacteria produced in food products, monitoring pollutant concentration levels, revealing the atmosphere of exoplanets, etc.
Just a year ago, this ISCR scientist and her team also initiated a collaboration with Jean-Marie Tarascon, a professor at the Collège de France, and an internationally renowned researcher in the field of energy storage. This new project aims to implement in situ monitoring of the chemical phenomena involved in the functioning of batteries, in an effort to improve their reliability and lifespan. “To access the chemistry of batteries while they are in operation, we opted for sensors that use chalcogenide glass optical fibres powered by a laser,” Boussard-Plédel adds. “Such sensors can detect the dynamic evolution of electrolytes in real time, as well as the products resulting from their decomposition.”

Integrated microsensors for tracking water pollution
A hundred kilometres north of Rennes, the FOTON Institute12 in Lannion also uses chalcogenide glass, but in the form of thin layers. These microscopic structures are made on the ISCR's premises, and are intended for applications in integrated optics, which uses similar technology as microelectronics to produce very small optical components.
As part of a recent ANR project led by Virginie Nazabal, a CNRS research professor at the ISCR – in collaboration with the French Geological Survey (BRGM) and IFREMER – the FOTON Institute embarked on the development of optical microsensors for in situ monitoring of freshwater and saltwater. “This involves designing a compact and portable device with a sensor consisting of an integrated waveguide in chalcogenide glass, as well as a quantum cascade laser emitting in mid-infrared,” explains Joël Charrier, who is an associate professor at the FOTON Institute.

To meet this challenge, the researcher can rely on the Lannion-based CCLO, a technological platform of the FOTON Institute entirely dedicated to the production and characterisation of integrated optical circuits. Once operational, this miniaturised detection device will be able to identify a wide range of aquatic pollutants ranging from volatile organic compounds to polycyclic aromatic hydrocarbons and nitrates. “Since the primary absorption range of aquatic pollutants is in the mid-infrared, almost all of these substances could be targeted by our optical sensors,” Charrier concludes.
- 1. In France this event will involve various professional and scientific organisations representing glass on the national level, including the French Union for Science and Glass Technology, which brings together researchers from academia, the glass industry, and glass artists.
- 2. Léon Brillouin “Diffusion de la lumière et des rayons X par un corps transparent homogène-Influence de l'agitation thermique”, Annales de physique, vol. 9, 1922, p 88-122.
- 3. This discovery, which was published by the Indian scientist Chandrashekhara Venkata Râman in 1928, was recognised with the Nobel Prize in Physics two years later.
- 4. Franche-Comté Electronics, Mechanics, Thermal Science and Optics – Sciences and Technologies (CNRS / Comue Université Bourgogne Franche-Comté).
- 5. CNRS / Université de Lille.
- 6. CNRS / Université de Lille.
- 7. CNRS / Aix-Marseille Université / Centrale Marseille.
- 8. CNRS / Université de Limoges.
- 9. Today the GRIFON GIS includes seven research centres: The Nice Institute of Physics (CNRS / Université Côte d’Azur), the PHLAM (CNRS / Université de Lille), the Xlim Institute (CNRS / Université de Limoges), the “glass and ceramics” team at the Rennes Institute of Chemical Sciences (CNRS / Université de Rennes 1 / École Nationale Supérieure de Chimie de Rennes), the Institute of Light and Matter (CNRS / Université Claude Bernard Lyon 1), the Carnot de Bourgogne Interdisciplinary Laboratory (CNRS / Comue Université Bourgogne Franche-Comté), and the Institute of Condensed Matter Chemistry of Bordeaux (CNRS / Institut Polytechnique de Bordeaux / Université de Bordeaux).
- 10. CNRS / Université Côte d’Azur.
- 11. CNRS / Université de Rennes 1 / École Nationale Supérieure de Chimie de Rennes / Insa.
- 12. CNRS / Université de Rennes 1.
Keywords
Share this article
Author
After first studying biology, Grégory Fléchet graduated with a master of science journalism. His areas of interest include ecology, the environment and health. From Saint-Etienne, he moved to Paris in 2007, where he now works as a freelance journalist.
Comments
Log in, join the CNRS News community