You are here
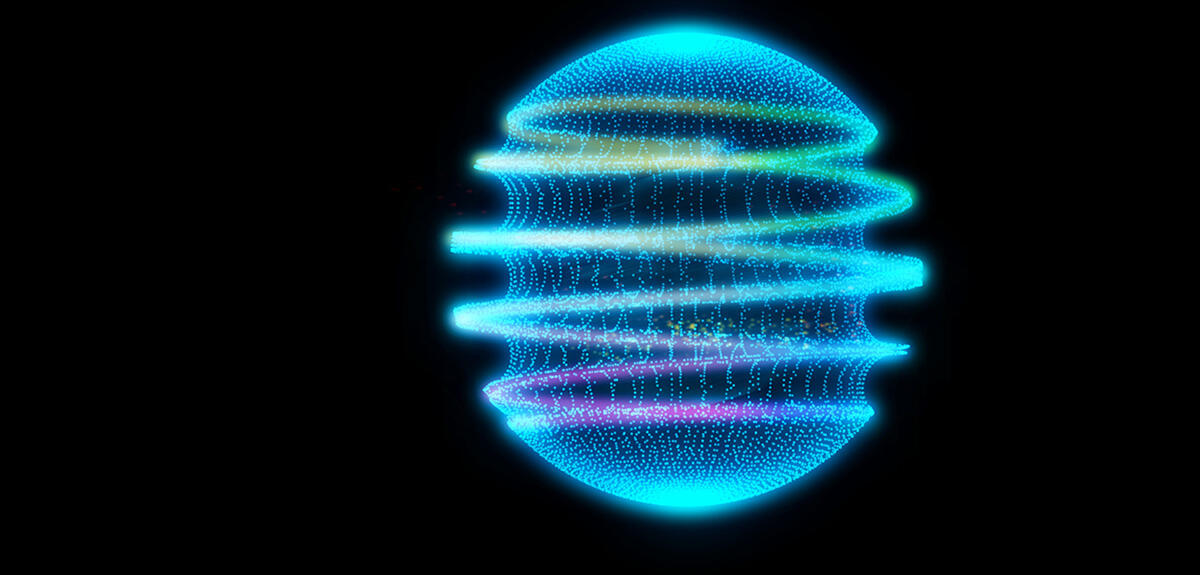
This is a journey that takes us to the crossroads of cosmology, astrophysics, and particle physics. It began in 1933 with an astrophysical observation: visible matter is not enough to account for the movement of stars in galaxies, or that of galaxies in galactic clusters. An invisible “dark” matter must be added, one that interacts very little with ordinary matter. Even though this component is thought to represent 84% of the total density of the matter in the Universe, it remains hypothetical today, its nature still shrouded in mystery. “While we do not know what dark matter is made of, we know it is certainly not something we are familiar with,” sums up Fabrice Hubaut, a CNRS research professor at the Marseille Particle Physics Centre (CPPM), in southeastern France1.
One of the candidates is a particle known as an “axion”, which had originally been proposed to solve an entirely different challenge in particle physics.
Restricted parameters
The Standard Model of particle physics is a theory that describes all known particles and their interactions: it has never been disproved by experiments, although its formulation contains a number of limitations. In particular, observations have constrained the value of certain parameters, with no underlying theoretical explanation. For example, in the 1970s it became clear that one of the theory’s parameters governing the structure and stability of atoms has an extremely small value.
This parameter has two contributions, one connected to the strong interaction that structures the nuclei of atoms, the other to the electroweak force, which combines electromagnetism and the force responsible for the radioactive decay of particles. “In the Standard Model, these two forces have no apparent connection. But in order for the parameter to be near zero, these two contributions have to exactly compensate each other,” points out Jérémie Quevillon, a CNRS theorist at the LPSC2.
Two physicists, Roberto Peccei of Italy and Helen Quinn of Australia, proposed a mechanism that solved this so-called “strong CP” problem. Steven Weinberg and Frank Wilczek, who would be awarded the Nobel Prize for Physics for other research, inferred the existence of a particle called an “axion” – from the name of a brand of laundry detergent, since its existence “cleared” the problem!
The axion, a serious candidate
And that’s not the end of the story. The particle modelled in this manner has very strong couplings with other particles in the Standard Model, and therefore could have been observed in the accelerators of the time. “This axion was excluded by the data back in the 1980s,” recounts Julien Lavalle, from the Montpellier Universe and Particles Laboratory (LUPM)3. “So the focus shifted to an invisible axion model, with a mechanism reducing these tricky couplings, all while preserving the properties that address the strong CP issue.” The axion subsequently became arbitrarily light, making it an excellent candidate for dark matter, with masses on the order of a millionth of a billionth of a proton’s mass. These properties, however, make it difficult to detect.
“Axions have been serious candidates for explaining dark matter for 40 years,” stresses Lavalle. Other particle candidates nevertheless exist. Weakly Interacting Massive Particles (WIMPs) in particular were proposed following a history similar to that of axions – namely based on another problem in particle physics – and have interesting properties for observation. Unlike axions, WIMPs were apparently more commonly produced from the primordial plasma at the birth of the Universe, just like ordinary matter. “Research initially concentrated on WIMPs, but no reliable observations have yet been made, resulting in renewed interest in axions today,” the physicist concludes. This is all the more true as technological advances now enable the potential discovery of dark matter in the form of axions.
Direct and indirect methods
When looking for an axion and its tiny mass, using a particle accelerator in the hopes of having the particle interact with an atom in a detector – a method widely used for WIMPs – does not work. However, this lightness also suggests that axions must be present in much larger numbers than WIMPs in order to have the same mass as dark matter. There would subsequently be hundreds of billions of particles per cubic centimetre, behaving more like waves than individual particles, which allows for considering other methods of detection.
The primary technique couples axions with photons, the elementary particles that carry light energy. This is “paradoxical”, Quevillon points out with a smile, “because by definition dark matter is not visible”. By applying a magnetic field, it would be possible to convert axions into photons, which can be studied using a device known as a haloscope. “A good haloscope requires three conditions to be met: a strong magnetic field, an environment that is resonant at very low temperatures (below 50 millikelvins) in order to avoid thermal noise, and good signal amplification,” indicates Quevillon.
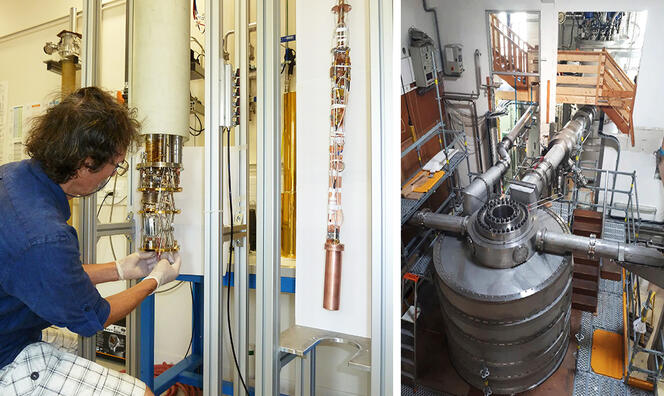

The laboratories located in Grenoble (southeastern France) boast world-renowned expertise in all three fields. The GrAHal (for Grenoble Axion Haloscopes) experiment based there is currently gathering initial data using an advanced prototype. A platform will eventually integrate numerous haloscopes functioning simultaneously. Resonant cavities are used for signal amplification, but they have a disadvantage: they must be set to the axion’s presumed mass. This either requires increasing the number of haloscopes, or performing constant adjustments in order to scan all possible masses.
“The idea of the MadMax experiment4, which I am in charge of in France, is to overcome these limitations,” explains Hubaut. This new type of resonator is based on a series of semi-transparent mirrors that allow the electromagnetic wave that is light to both pass through and to reflect: by superimposing the waves in this way, the signal is amplified. To test multiple masses, the mirrors simply need to be moved in relation to one another.
“We are still in an R&D phase, with many challenges at all levels: the mirrors have to be smooth, the magnetic field intense, the equipment for moving the mirrors very precise, and all this at very low temperatures,” warns the physicist. MadMax is one of the experiments being developed within the Dark Matter Lab, which was officially launched in June 2023. This International Research Laboratory brings together the CNRS and three of Germany’s most important and prestigious research institutions5. It will test multiple detection methods for the possible components of dark matter.
Meanwhile, at the LAPTh6 in Annecy-le-Vieux (southeastern France), Francesca Calore studies the signatures of axion-like particles culled from high-energy astrophysics data. This is essentially an indirect approach using information collected by land-based or space telescopes. “We are looking for unexplained signals that could point to the conversion of axions into photons, for example in our galaxy’s halo, where dark matter should be present,” explains Calore, who is also interested in the scenario in which axions exist, but are not part of the composition of dark matter.
The mystery remains
Other experiments, for instance, seek to find axions that could be produced within the Sun’s core, or during the explosive final phase of the lives of massive stars (supernovae). “The difficulty resides in completely and precisely understanding all of the other astrophysical phenomena within the collection of data, in order to isolate a genuine signal. It is a fascinating theoretical and technical challenge!” the scientist says.
To date, neither direct nor indirect research – nor the few laboratory experiments attempting to produce axions from photons (with coupling functioning in both directions) – have yielded results. However, each trial limits the range of possible parameters, and imposes constraints on the model for the axion particle. “I am agnostic, in both life and research,” confides Calore. “While the theoretical motivations for axions are highly convincing, we must keep in mind that they could potentially not make up dark matter, or not even exist at all. But we do have interesting data that must be explored.”
“Conducting a test that has never been tried before, even if the result is negative, remains a major advance, for we are creating knowledge,” confirms Hubaut, who stresses that dark matter might just as well be primordial black holes, modified gravity, or a combination of various types of particles that are already known, or have yet to be conceptualised. It remains a mystery, but the journey continues, as scientists are considering other experiments. Dark matter still makes their eyes shine. ♦
- 1. CNRS / Aix-Marseille Université.
- 2. Laboratoire de Physique Subatomique et de Cosmologie (CNRS / Université Grenoble Alpes).
- 3. CNRS / Université de Montpellier.
- 4. For MAgnetized Disc and Mirror Axion eXperiment.
- 5. Deutsches Elektronen-Synchrotron (DESY – German electron synchrotron), Gesellschaft für Schwerionenforschung mbH (GSI – centre for heavy-ion research), Karlsruher Institut für Technologie (KIT – Karlsruhe Institute of Technology,).
- 6. Laboratoire d'Annecy-le-Vieux de physique théorique (CNRS / Université Savoie Mont Blanc).
Comments
Log in, join the CNRS News community